
Stem Disease
Bot Canker
Botryosphaeria spp.
Diplodia spp.
Neofusicoccum spp.
and related genera
Botryosphaeria dieback, Esca, Eutypa dieback, and Phomopsis dieback make up a complex of “trunk diseases” caused by different wood-infecting fungi.
Location: California
Impact Significance:
Botryosphaeria dieback is the most common and widespread trunk disease in California and some of the causal species (e.g., Neofusicoccum parvum) are among the most aggressive trunk pathogens.
Hosts: oak trees, grape, giant sequoia, alder, coast redwood, incense cedar, madrone, tan oaks, many other woody species.
Biology:
Species in the fungal family Botryosphaeriaceae, including Neofusicoccum parvum, Diplodia seriata,and Lasiodiplodia theobromae.
Damage:
Botryosphaeria dieback (commonly known in California as “Bot canker”) causes death of spurs, arms, cordons, canes, and sometimes the upper section of the trunk, depending on the location of the wood canker.

Bot canker

Bot canker
Learn More
Acute Oak Decline
Brenneria goodwinii
Gibbsiella quercinecans
Rahnella victoriana
Lonsdalea britannica
Oak ‘decline’ is used to describe poor health in oak trees. Many living and non-living factors can cause oak decline and they can vary by location. Acute Oak Decline (AOD) is uniquely caused by multiple bacteria species. The disease has been occurring in the United Kingdom for many years, where trees die within 4-5 years after the first symptoms are found. It was detected on a single black oak tree in San Diego in 2010 by UK researcher Dr. Sandra Denman and was recently found in Hollister, California in 2023. Since then, AOD has been detected in multiple locations throughout the state.
Location: California
Impact Significance: Oak woodlands support higher levels of biodiversity than any other terrestrial ecosystem in California. In addition to providing important habitats fomr wildlife, oaks are frequently used landscaping purposes and hold enormous cultural and economic value. The complexity of the cause of AOD and the importance of the tree species affected give cause for concern.
Hosts: AOD attacks 11 oaks species in the UK. In California, it has been found on black oak (Quercus kelloggii), coast live oak (Quercus agrifolia) and blue oak (Q. douglasii).
Symptoms:
The main symptom is bleeding of a dark, sticky fluid from small lesions or splits in the bark. These symptoms have mainly been seen at ground level but can be observed further up the trunk of the tree (1-2m) as well.
The bark splits longitudinal and form cracks between the bark plates. They occur close to each other and at the bleeding point, underneath the outer bark, the inner bark breaks down, leaving a fluid filled cavity. In some cases, signs of callusing can be seen in an attempt by the tree to heal the cavity.
Trees may show chlorotic leaves, thinning and dieback of the canopy. This can be severe and may not occur until the tree is near death.
In the UK, AOD has been associated with a wood boring species, Agrilus biguttatus. This species has not been found in the US, however AOD has been found on a few trees that have been attacked by GSOB.
Management
There is no cure for acute oak decline, but there are some management recommendations:
-
Leave infected trees in place and continue to monitor, unless immediate concern about safety.
-
Minimize any contact with bleeds to reduce transferring the disease from tree to tree.
-
If only a limited number of trees appear to be infected on a site, it may be prudent to fell and destroy the infected individuals to keep infection levels low and reduce risk of infecting healthy trees.
-
Avoid felling when conditions are wet to reduce chance of spreading the pathogens.
-
Don't move logs and branches off site after felling.
-
Don't use bark chips or other parts of infected trees (e.g. leaves, bark, wood) for mulch, compost of soil conditioner.
-
It is unknown how long the bacteria can survive in affected wood, so not moving infested material to a new location is important.
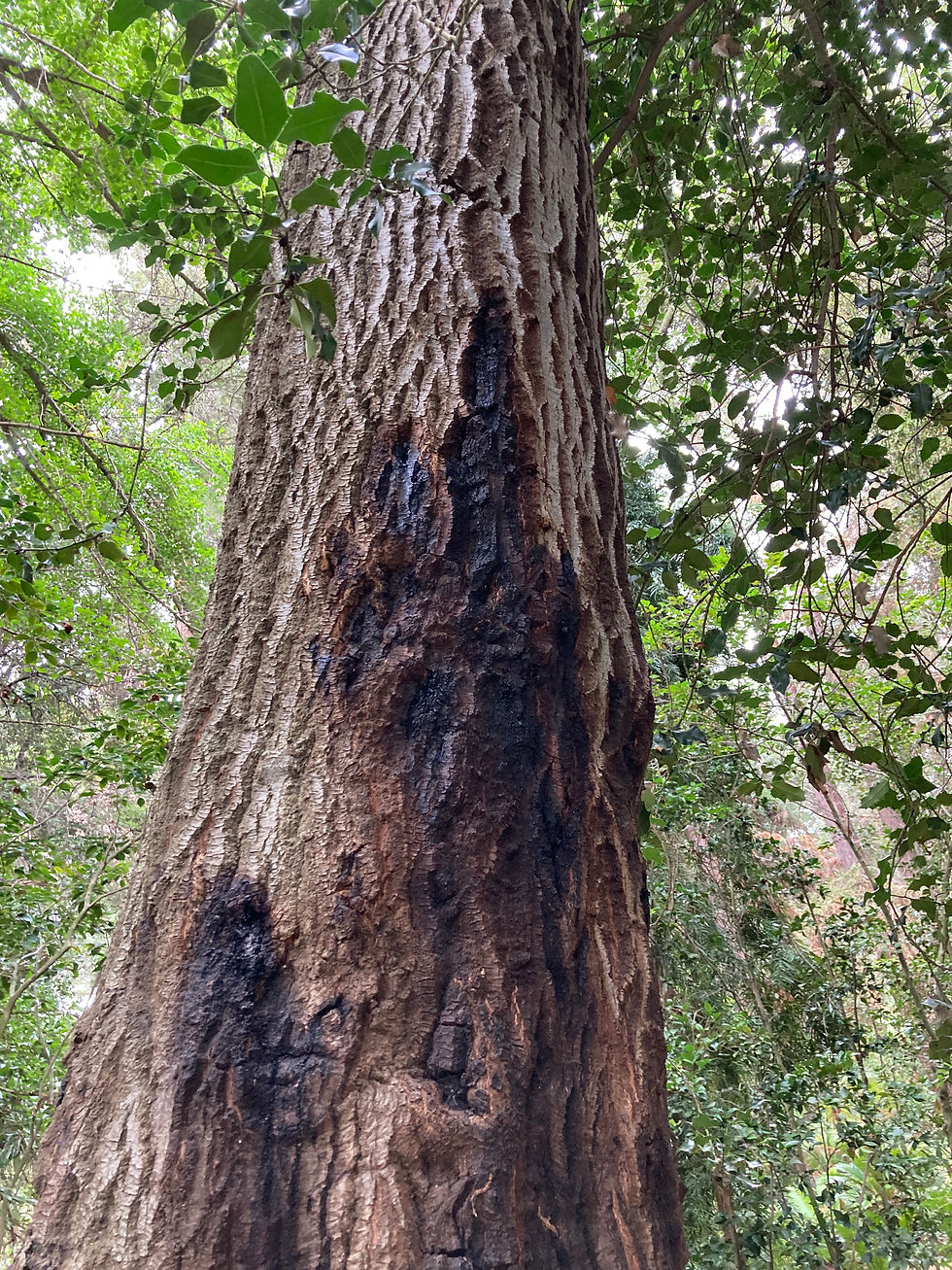
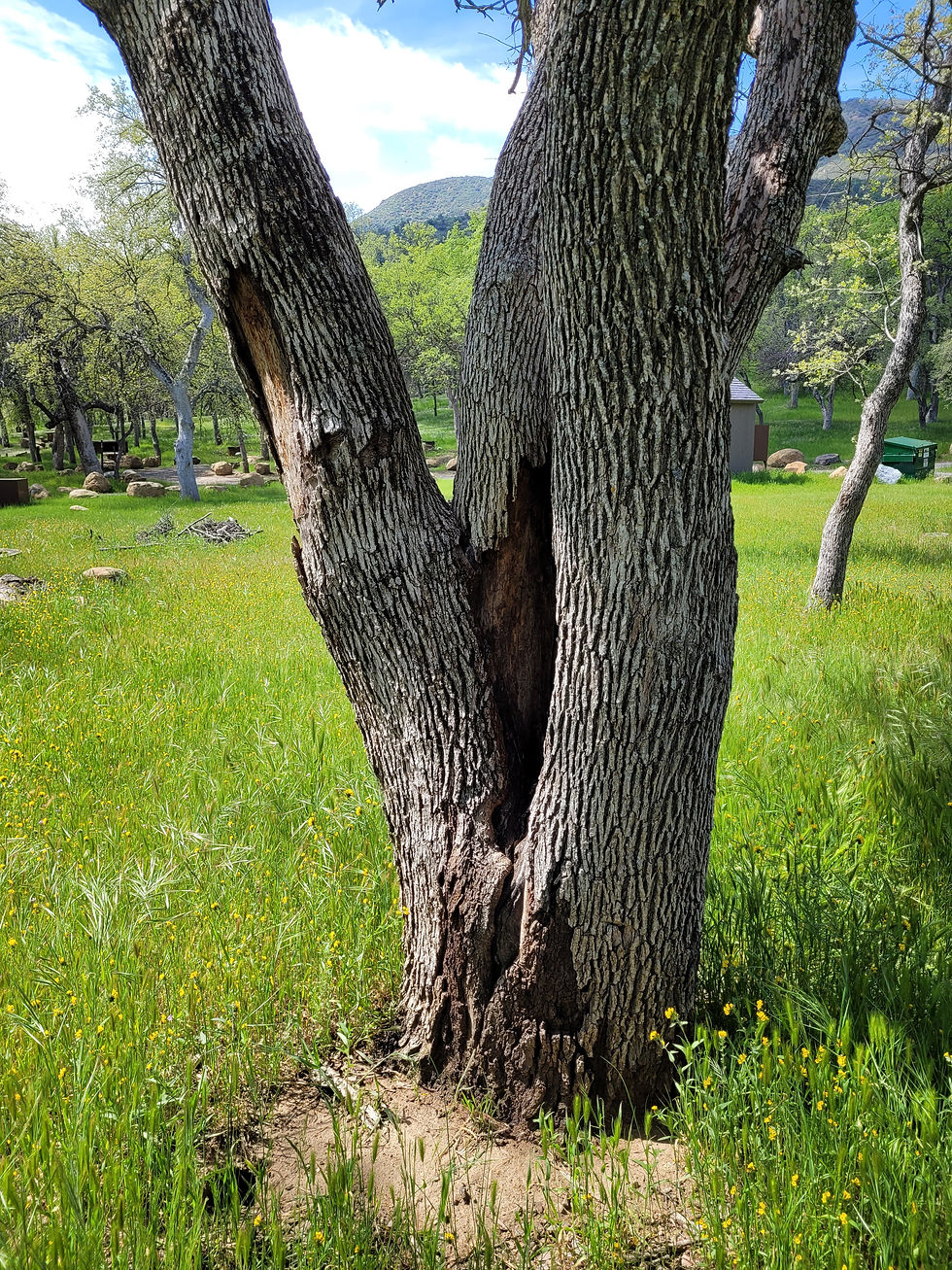

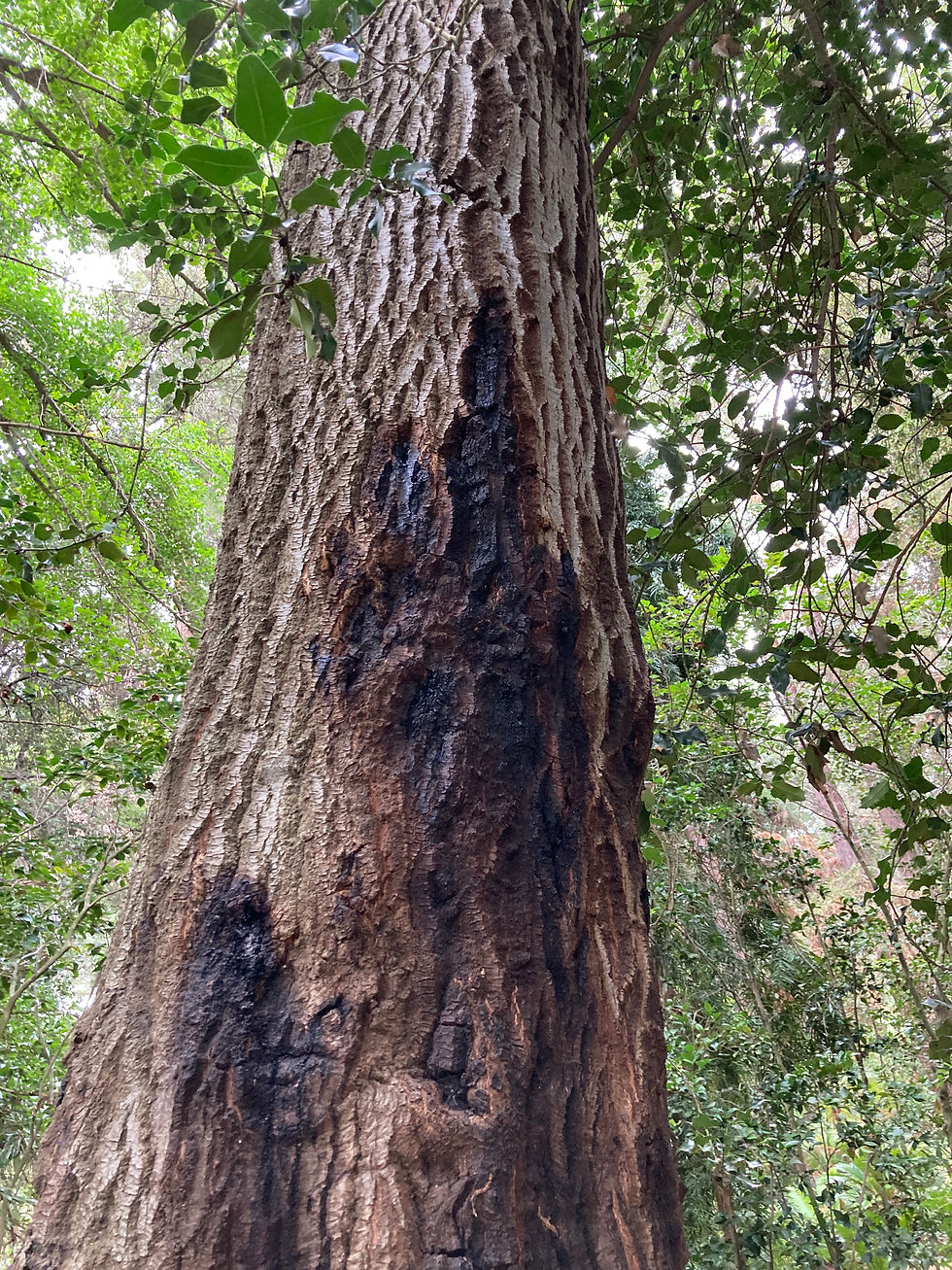
Keep your oak trees healthy and prevent spreading this disease by:
-
Sanitize any tools between trees to prevent spread.
-
Sanitize with Lysol® spray, a 70% or greater solution of isopropyl alcohol, or a Clorox® solution (1-part Chlorox® to 9 - parts water or Clorox Cleanup®). Remember that these products are corrosive to metal and fabric. Rinse your gear after sanitation.
-
Avoid contact with trees showing symptoms such as stem bleeds and dead or dying branches.
-
Do not touch the bleeding areas on tree stems.
-
If you have been walking or cycling in an area where there are diseased trees, clean soles of walking shoes and bicycle tires before entering areas where trees are not yet affected.
-
Do not remove dead wood, branches or infected bark from the wooded area.
Reporting
-
Please report to your local Agricultural Commissioner or UC Cooperative Extension Office if suspected or found, https://www.cdfa.ca.gov/exec/county/countymap/
Learn More
Chestnut Blight
Cryphonectria parasitica
Chestnut blight, or chestnut bark disease, is caused by an introduced non-native fungus, Cryphonectria parasitica (Murrill) Barr, (formerly Endothia parasitica [Murrill] Anderson & Anderson). The fungus enters wounds, grows in and under the bark and eventually kills the cambium all the way around the twig, branch, or trunk.
Location: eastern US, Europe, SE Asia
Impact Significance: This introduced fungus enters wounds, grows in and under the bark and eventually kills the cambium all the way around the twig, branch or trunk. Sprouts develop from the root collar and those will also become wounded and infected, and die, and the process starts all over again. None of the control attempts against this pathogen were successful and the iconic American chestnut tree throughout its native range have been reduced to a multiple-stemmed shrub.
Hosts:
Species in the genus Castanea (Family Fagaeceae), particularly the American Chestnut (C. dentata), European Chestnut (C. sativa). Minor hosts include oaks (Quercus spp.), maples (Acer spp.) European hornbeam (Carpinus betulus) and American chinkapin (Castanea pumila).
Disease Cycle: Conidia and ascospores are carried by eddies of wind to new hosts or infect other parts of the same tree. When insects, birds, or other wildlife come in contact with the cankers, they can mechanically transfer the conidia to new hosts. These spores then infect wounds and fissures in the outer bark. The fungus grows in the inner bark and cambium, producing small brownish mycelial fans. The cankers girdle the stem, severing the flow or nutrients and water to the vital vegetative tissues. Even after the branch or stem is girdled and killed the fungus continues to colonize it, producing ever more inoculum. Once the above ground portion of the tree is dead the root system will survive and as a result American chestnuts can re-sprout. These sprouts then usually succumb to infection before reaching sexual maturity.
Management:
Hypovirulence - Most management attempts have failed to control chestnut blight, tree removal, burning infected trees, etc., however, the mycovirus Cryphonectria hypovirus 1 (CHV-1) infects C. parastica and reduces its parasitic growth and sporulation capacity. This hypovirulence is one management method though it has limitations and has shown promise in locations such as West Salem Wisconsin and Michigan in the United States and some locations in Europe where chestnuts were planted outside their natural range. This treatment has almost completely failed in eastern North America.
Breeding for resistance - Chinese or Japanese chestnut are somewhat resistant to chestnut blight. Researchers have identified two or three genes that allow for blight resistance and are focusing on giving the American chestnut hybrids only those genes from the Chinese chestnut (Castanea mollissima) or Japanese chestnut (Castanea crenata). The two species are first bred to create a 50/50 hybrid. After three back crosses with American chestnut, the remaining genome is approximate 1/16 that of the resistant tree, and 15/16 American. The strategy is to select blight-resistance genes during the back crossing, while preserving the more wild-type traits of American chestnut as the dominant phenotype. Thus, the newly bred hybrid chestnut trees should reach the same heights as the original American chestnut. Many of these 15/16 American chestnut hybrids have been planted along the East Coast, including in the Jefferson National Forest and on the Flight 93 National Memoria.
Transgenic blight-resistant chestnut trees - A 1983 study on hypovirulence shown that chestnut blight infected with hypovirus produced less oxalic acid when attacking the cambium. Meanwhile, a plant pathologist, Dr. William Powell, had been trying to figure out how to transfer all of the Asian chestnut's resistance genetics to its American relatives. Then in the 1990s he had the idea to give up on the more complicated and larger job, and instead look around for a single gene elsewhere. (In related work, in 2001 Liang, Mayard, Allen, and Powell successfully inserted an oxalate oxidase (OxO) gene from wheat into Populus × euramericana ("Ogy") for Septoria musiva resistance. This enzyme breaks down the oxalic acid secreted by the fungus into carbon dioxide and hydrogen peroxide.) In 2007 Welch, Stipanovic, Maynard, and Powell showed that transgenic C. dentata expressing a wheat OxO did indeed have lower lignin degradation by OA, and suggested this was the path to take.
A few years later this line of research culminated in the final product: Powell and another plant path - Dr. Charles Maynard - working at the State University of New York College of Environmental Science and Forestry (SUNY ESF) have developed American chestnuts which have full blight resistance. Full resistance was attained by introducing a wheat OxO gene into the American chestnut genome. The transgenic trees have blight resistance either equal to or surpassing that of Chinese chestnuts. In 2013, SUNY ESF had over 100 individual events being tested, with more than 400 slated to be in the field or in the lab for various assay tests in the next several years and more than 1,000 trees growing in several field sites in 2014.Government approval will be required before returning any of these blight resistant trees to the wild. The New York Botanical Garden has planted several of the transgenic trees for public display.
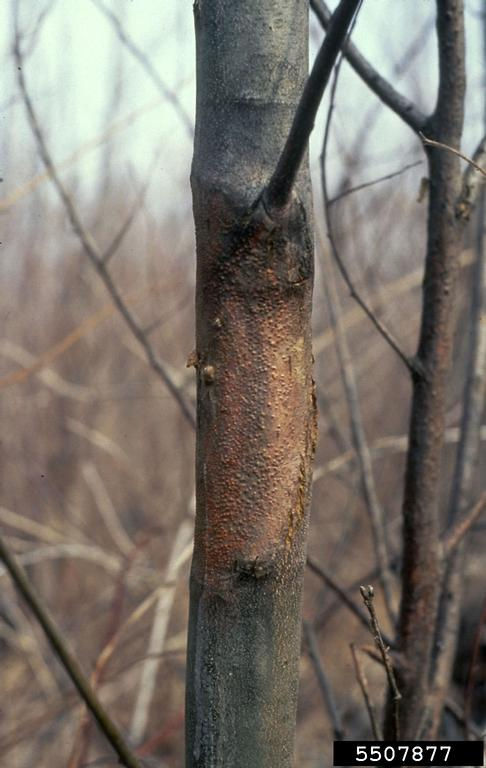
Chestnut blight
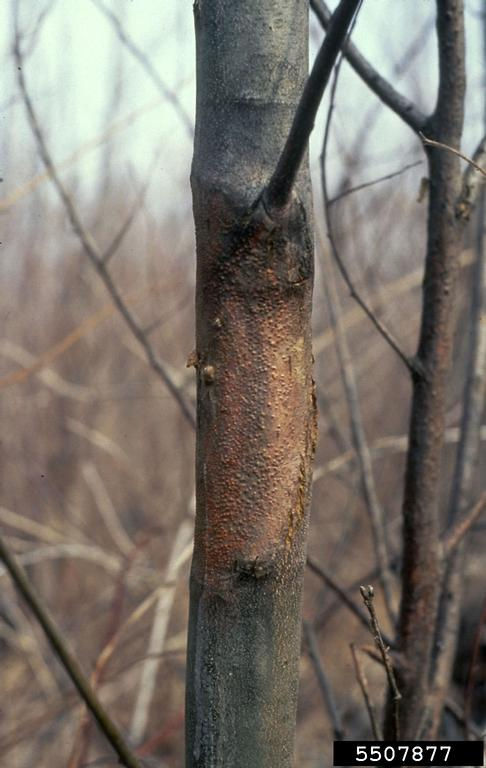
Chestnut blight
Learn More
Fusarium Dieback - Invasive Shothole Borers
Fusarium euwallacea - Euwallacea fornicatus
F. kuroshium - E. kuroshio
Status: Introduced
A pest-pathogen complex caused by two closely related ambrosia beetle species that form a symbiotic relationship with specific pathogenic fungal species of Fusarium and other fungi. Fusarium kuroshium and Fusarium euwallaceae are tree diseases caused by the pathogenic fungi of the genus Fusarium. The invasive shot hole borers are dependent on the fungi it carries for survival. The dispersing female carry's spores of symbiotic fungi in its specialized structures called mycangia. As the female beetle bores into the tree to create the galleries, the fungi introduced and grows inside the galleries and serves as the sole food source for both larvae and adults. Once established, the fungi colonizes the wood beyond the gallery wall and blocks the water movement in the trees vascular system, causing stress and dieback.
Fusarium dieback symptoms include pale and wilted leaves and branch dieback. The beetle entry holes are often surrounded by wet discoloration, gumming, or white powdery exudate. Scraping off the bark will reveal a brown elliptical shape discoloration surrounding the beetle galleries.
For more information, see Invasive Shothole Borers.

Adult beetle on trunk of tree

ISHB adults in gallery on oak


Adult beetle on trunk of tree
Laurel Wilt
Raffaelea lauricola and Xyleborus glabratus
Status: Introduced
A systemic vascular wilt disease caused by the ophiostomatoid fungal pathogen Raffaelea lauricola and the redbay ambrosia beetle (Xyleborus glabratus), its primary vector.
Location:
Southeastern US. Link to full distribution map here.
Hosts:
Members of the Lauraceae family, including avocado (Persea americana). Severely impacted tree species native to the southeastern United States include redbay (Persea borbonia), silk bay (P. humilis), swamp bay (P. palustris), sassafras (Sassafras albidum), pondspice (Litsea aestivalis), and pondberry (Lindera melissifolia). Although the disease is currently not in California, artificial inoculation experiments confirmed that California bay laurel (Umbellularia californica) is highly susceptible (Fraedrich 2008). Other hosts not native to but present in California include avocado, camphortree (Cinnamomum camphora) and sweet bay (Laurus nobilis) (Smith et al. 2009; Hughes et al. 2014).
Management:
Management options to control laurel wilt are limited in the southeastern United States, where the beetle-pathogen has established and can no longer be eradicated. Thus, preventing its introduction into California is critical to protecting the 55,000 acres of avocado production and 1.2 million acres of hardwood and conifer forests that harbor California bay laurel throughout the state. Prevention involves controlling and containing the pest in the southeast, where it has spread across 11 states since 2002 (Olatinwo et al. 2021). Monitoring of sentinel trees along potential routes of introduction should also be a priority. Within California, prevention also entails risk assessments of priority sites and early detection monitoring to elicit a rapid response in the event of a novel introduction. Trapping is a useful tool for monitoring and should be used to detect the primary vector (X. glabratus) and potential alternate vectors (within Xyleborus and Xyleborinus), but is not an effective control treatment. The standard ethanol lures for ambrosia beetle detection can be used for trapping alternate vectors, but lures with an essential oil product enriched to 50% (–)-α-copaene content must be used to attract X. glabratus (Kendra et al. 2016). Consult your local county agricultural commissioner and forest health professional if you observe signs and symptoms on a suspect tree (see below). Because the beetle-pathogen can live in cut wood and human movement of firewood drives long-distance spread of laurel wilt (Ploetz et al. 2017), it is imperative not to move firewood over long distances. Instead, buy locally or gather on site where permitted.
Early detection, rapid removal, and destruction of affected trees is the only measure proven to be effective at slowing disease spread in the southeast, especially in commercial avocado orchards. Stumps of felled infested trees are ground to soil level, and all wood is chipped to at least 1 square inch using sterilized equipment, followed by onsite burning, insecticide spray, or solarization for an extended time in a designated space (Crane et al. 2020). Prophylactic Tilt® injections (the fungicide propiconazole) have been shown to prevent laurel wilt outbreaks in commercial avocado groves (Crane et al. 2020), and could be an effective short-term measure on high-value trees or non-symptomatic hosts adjacent to infested trees. However, the treatment is very expensive, has a slow uptake rate, and needs to be reapplied every 12–24 months, depending on the dose, tree size, and onsite disease status at the time of injection (Crane et al. 2020, Olatinwo et al. 2021). Trenching or fungicide/herbicide treatments to sever root grafts may slow disease spread in avocado orchards where the pathogen can spread from tree to tree without vector assistance via interconnected root systems (Ploetz et al. 2017). Research is underway on treatment options involving host genetic resistance or tolerance and biocontrol agents (i.e., endophytic fungi and bacteria, extracts from the secondary metabolites of fungi, and entomopathogenic fungi).
Highlights:
Laurel wilt disease is a pest-pathogen complex introduced into the southeastern United States from Southeast Asia. Since 2002, the redbay ambrosia beetle vector (Xyleborus glabratus) and fungal pathogen (Raffaelea lauricola) have killed an estimated 300 million redbay trees and other native lauraceous species in urban-wildland forests throughout the region (Ploetz et al. 2017). The disease has also significantly impacted the avocado industry in Florida. Growers can lose up to 15-20 trees per month (Crane et al. 2019), with up to $356 million estimated in potential economic losses (Evans et al. 2010). The complex is unique for two reasons: 1) unlike most ambrosia beetles, the redbay ambrosia beetle prefers to attack healthy, non-stressed trees; 2) R. lauricola aggressively moves systemically through the host xylem, unlike most ambrosia fungal symbionts, which cause local infections. As few as 100 conidia of R. lauricola can kill avocado and swamp bay (Hughes et al. 2015a). Tolerant host species, such as camphortree, could serve as symptomless reservoirs of the beetle-pathogen in the landscape and play a key role in disease persistence. Interestingly, R. lauricola has been horizontally transferred from the redbay ambrosia beetle to nine additional ambrosia beetle species since its introduction (Carrillo et al. 2014; Ploetz et al. 2017). These other species can also transmit the pathogen (Carrillo et al. 2014), suggesting potential for disease to spread among native and non-native beetle species if it were introduced in California. For avocado, the other Southeast native Xyleborus species (X. bispinatus and X. volvulus) are more important vectors of the pathogen than the redbay ambrosia beetle (Carrillo et al. 2014; Cruz et al. 2018, 2021, 2019; Saucedo-Carabez et al. 2018).
In the early stages of the epidemic, adult female ambrosia beetles attack large healthy trees, attempting to bore natal galleries. Galleries are aborted, but trees are inoculated with the pathogen in these initial attacks, and the fungus spreads quickly throughout the tree’s vascular system (Fraedrich et al. 2008, 2015). Fungal propagules are also dispersed on other beetle species’ exoskeleton (Cruz et al. 2021), which may be sufficient for pathogen transmission. Once disease develops, infected host trees increase production of volatiles that attract more beetles, promoting a mass attack, colonization, and brood development within galleries of the host xylem where the developing colony feeds on the fungal symbiont cultivated in the gallery wall (Fraedrich et al. 2008, 2015). The host responds to attack in as little as three days before visible symptoms develop by producing tyloses and gels that block the xylem. Symptoms of discolored black sapwood and wilting green leaves that turn brown and persist on dying branches develop, and small holes with sawdust “toothpicks” are visible where the ambrosia beetles bore into the tree. Within a few weeks to several months, trees become choked of water and die. The pathogen can “silently” spread in avocado orchards where root-to-root transmission is common, with trees remaining asymptomatic until the epidemic is at the advanced stage. Males remain inside their natal galleries while mature adult females emerge carrying spores of R. lauricola in mycangia. The redbay ambrosia beetle uses host olfactory and visual cues in flight (not sex aggregation pheromones) to find a new host and produce their new gallery. Once laurel wilt establishes in a stand, most mature trees are killed within a few years.
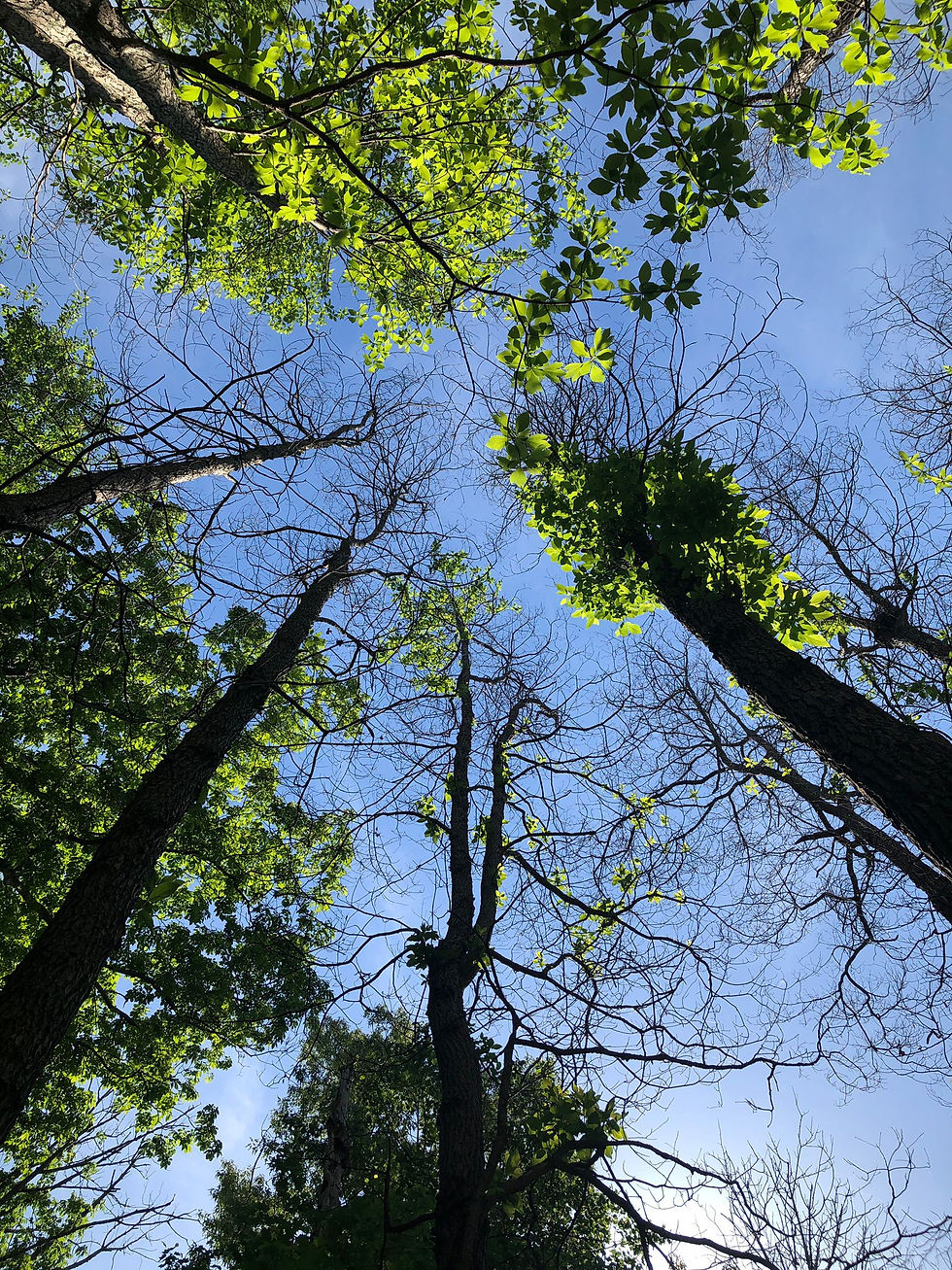
Laurel wilt disease-induced sassafras (Sassafras albidum) mortality in Cherokee Park in Morristown, Tennessee. Photo: Romina Gazis, University of Florida Tropical Research and Education Center.
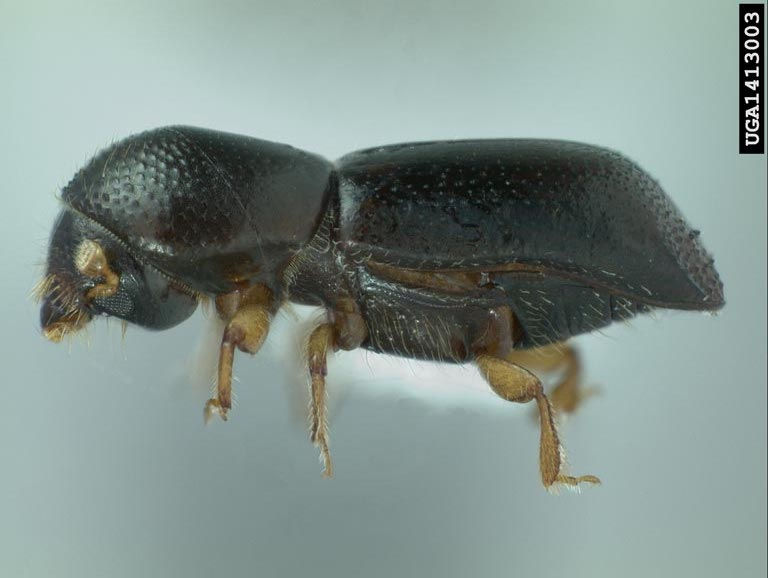
Redbay ambrosia beetle (Xyleborus glabratus).

Laurel wilt
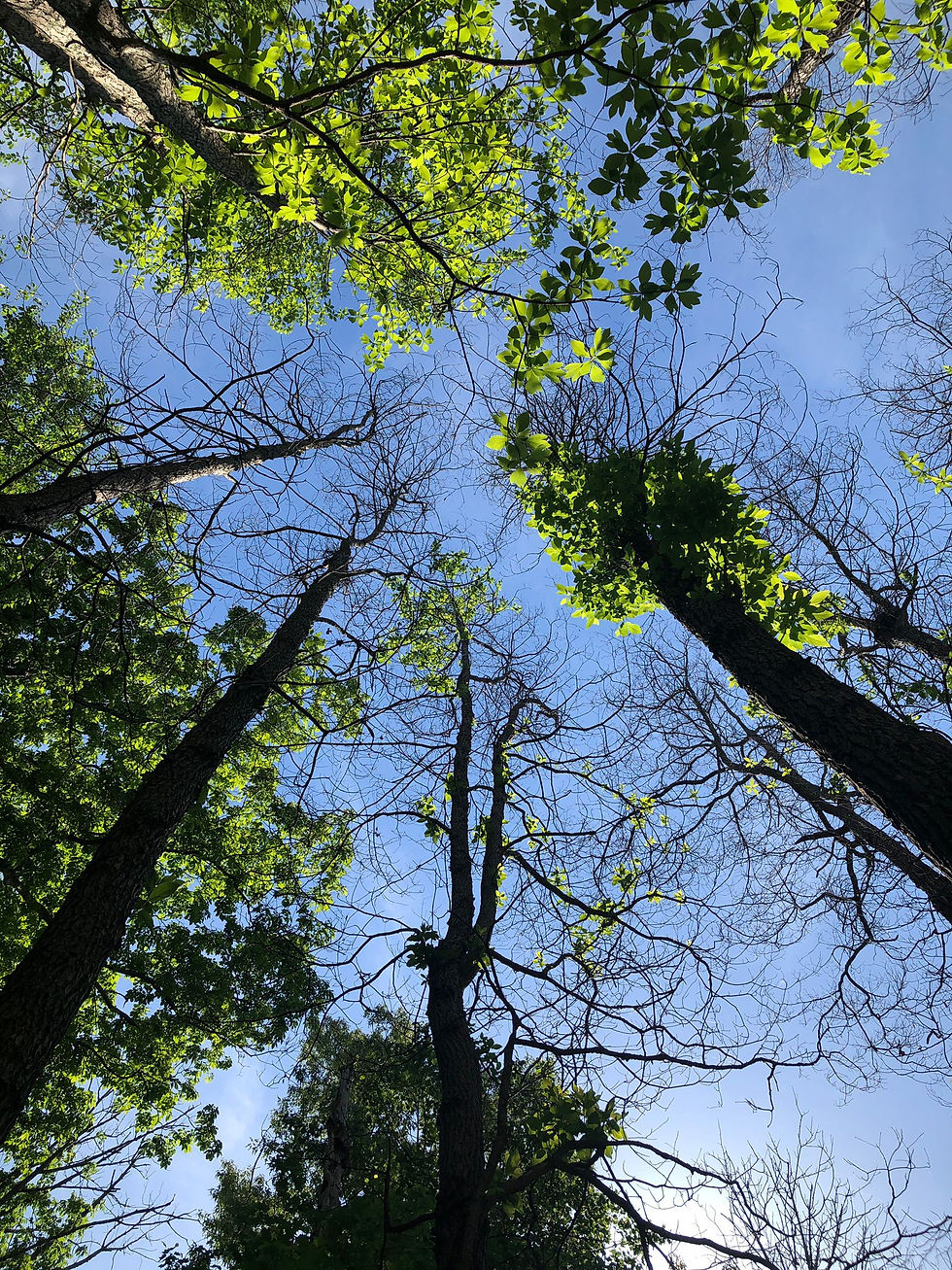
Laurel wilt disease-induced sassafras (Sassafras albidum) mortality in Cherokee Park in Morristown, Tennessee. Photo: Romina Gazis, University of Florida Tropical Research and Education Center.
Learn More
Recommended Literature
Carrillo, D., Duncan, R. E., Ploetz, J. N., Campbell, A. F., Ploetz, R. C., and Peña, J. E. 2014. Lateral Transfer of a Phytopathogenic Symbiont Among Native and Exotic Ambrosia Beetles. Plant Pathology. 63:54–62. http://dx.doi.org/10.1111/ppa.12073.
Crane, J. H., Carrillo, D., Evans, E. A., Gazis, R., Schaffer, B. A., Ballen Orozco, F. H., et al. 2020. Recommendations for Control and Mitigation of Laurel Wilt and Ambrosia Beetle Vectors in Commercial Avocado Groves in Florida. EDIS. 2020. http://dx.doi.org/10.32473/edis-hs1360-2020.
Crane, J.H., J. Wasielewski, D. Carrillo, E.A. Evans B. Schaffer, F. Ballen, and R. Gazis. 2019. Effect of the Laurel Wilt Epidemic on Florida’s Avocado Industry. IX World avocado Congress. Medellín, Colombia.
Cruz, L. F., Menocal, O., Kendra, P. E., and Carrillo, D. 2021. Phoretic and Internal Transport of Raffaelea lauricola by Different Species of Ambrosia Beetle Associated with Avocado Trees. Symbiosis. https://doi.org/10.1007/s13199-021-00776-2.
Cruz, L. F., Menocal, O., Mantilla, J., Ibarra-Juarez, L. A., and Carrillo, D. 2019. Xyleborus volvulus (Coleoptera: Curculionidae): Biology and Fungal Associates. Applied and Environmental Microbiology. 85. http://dx.doi.org/10.1128/aem.01190-19.
Cruz, L. F., Rocio, S. A., Duran, L. G., Menocal, O., Garcia-Avila, C. D. J., and Carrillo, D. 2018. Developmental Biology of Xyleborus bispinatus (Coleoptera: Curculionidae) Reared on an Artificial Medium and Fungal Cultivation of Symbiotic Fungi in the Beetle’s Galleries. Fungal Ecology. 35:116–126. http://dx.doi.org/10.1016/j.funeco.2018.07.007.
Evans, E. A., Crane, J., Hodges, A., and Osborne, J. L. 2010. Potential Economic Impact of Laurel Wilt Disease on the Florida Avocado Industry. Horttechnology. 20:234–238.
Fraedrich, S. W. 2008. California Laurel Is Susceptible to Laurel Wilt Caused by Raffaelea lauricola. Plant Disease. 92:1469–1469. http://dx.doi.org/10.1094/pdis-92-10-1469a.
Fraedrich, S. W., Harrington, T. C., and Best, G. S. 2015. Xyleborus glabratus Attacks and Systemic Colonization by Raffaelea lauricola Associated with Dieback of Cinnamomum camphora in the Southeastern United States. Forest Pathology 45(1):60-70.
Fraedrich, S. W., Harrington, T. C., Rabaglia, R. J., Ulyshen, M. D., Mayfield, A. E., 3rd, Hanula, J. L., et al. 2008. A Fungal Symbiont of the Redbay Ambrosia Beetle Causes a Lethal Wilt in Redbay and Other Lauraceae in the Southeastern United States. Plant Disease 92:215–224.
Hughes, M. A., Black, A., and Smith, J. A. 2014. First Report of Laurel Wilt Caused by Raffaelea lauricola on Bay Laurel (Laurus nobilis) in the United States. Plant Disease 98:1159.
Hughes, M. A., Inch, S. A., Ploetz, R. C., Er, H. L., van Bruggen, A. H. C., and Smith, J. A. 2015a. Responses of Swamp Bay, Persea palustris, and Avocado, Persea americana, to Various Concentrations of the Laurel Wilt Pathogen, Raffaelea lauricola. Forest Pathology 45:111–119.
Hughes, M. A., Smith, J. A., Ploetz, R. C., Kendra, P. E., Mayfield, A. E., Hanula, J. L., et al. 2015b. Recovery Plan for Laurel Wilt on Redbay and Other Forest Species Caused by Raffaelea lauricola and Disseminated by Xyleborus glabratus. Plant Health Progress. 16:173–210.
Hughes, M.A., Smith, J.A., and Coyle, D.R. 2016. Biology, Ecology, and Management of Laurel Wilt and the Redbay Ambrosia Beetle. Southern Regional Extension Forestry, Forest Health (SR-EF-006):1-8.
Kendra, P. E., Montgomery, W. S., Deyrup, M. A., and Wakarchuk, D. 2016. Improved Lure for Redbay Ambrosia Beetle Developed by Enrichment of α-Copaene Content. Journal of Pest Science. 89:427–438.
Ploetz, R. C., Kendra, P. E., Choudhury, R. A., and Rollins, J. A. 2017. Laurel Wilt in Natural and Agricultural Ecosystems: Understanding the Drivers and Scales of Complex Pathosystems. Forest Trees and Livelihoods. https://www.mdpi.com/1999-4907/8/2/48.
Saucedo-Carabez, J. R., Ploetz, R. C., Konkol, J. L., Carrillo, D., and Gazis, R. 2018. Partnerships Between Ambrosia Beetles and Fungi: Lineage-Specific Promiscuity Among Vectors of the Laurel Wilt Pathogen, Raffaelea lauricola. Microbial Ecology. 76:925–940. http://dx.doi.org/10.1007/s00248-018-1188-y.
Smith, J. A., Mount, L., Mayfield, A. E., Bates, C. A., Lamborn, W. A., and Fraedrich, S. W. 2009. First Report of Laurel Wilt Disease Caused by Raffaelea lauricola on Camphor in Florida and Georgia. Plant Disease 93:198–198.
Oak Wilt
Ceratocystis fagacearum
Location: Midwestern and Eastern US & Texas
Impact Significance: Oak wilt is a non-native and one of the most destructive tree diseases in the United States and kills oaks in epidemic proportions. Oak wilt is an infectious disease which invades and disables the water-conducting system (xylem) in oaks. All oaks can be infected by the fungus that causes oak wilt; however, some oak species are affected more than others. The successful management of oak wilt depends on correct diagnosis and an understanding of how the pathogen and different oak species interact.
Hosts: All Quercus spp. appear to be susceptible, with red oaks displaying more severe symptoms.
Disease Cycle:
The Oak Wilt fungus is spread by two ways: above ground and below ground.
The above ground movement is facilitated by a nitidulid beetle that carries the fungal spores to fresh wounds on healthy trees. The spores are produced on fungal mats that form beneath the bark of certain diseased red oaks in spring, but do not form on white or live oaks. Individual fungal mats produce spores for only a few weeks. The fruity odor of fungal mats attracts many kinds of insects, the most important of which are sap-feeding nitidulid beetles. The fungus is transmitted by these small beetles as they emerge from mats and visit fresh wounds on healthy oaks. Fungal mats are most commonly formed on standing trees, but they also can develop on logs, stumps, and fresh firewood cut from diseased red oaks. Red oaks play a key role in the establishment of new infection centers. The oak wilt fungus is spread overland by insect vectors and by humans through movement of wood from infected red oaks to other locations.
The below ground movement occurs when the fungus travels from tree to tree through interconnected roots. Live oaks tend to grow in large, dense groups (called motts) with interconnected roots. The fungus is transmitted from one tree to another through these root connections. As a result, patches of dead and dying trees (infection centers) are formed. Infection centers among live oaks in Texas expand at an average rate of 75 ft per year, varying from no spread to 150 ft in any one direction. Occasionally, the oak wilt fungus is transmitted through connected roots between other oak species, called grafted roots, but movement through grafted roots is slower and occurs over shorter distances than in live oaks.
Symptoms result from the fungal spores clogging the xylem vessels, causing tyloses formation and preventing water and nutrient flow.
Top cycle: Overland spread (initiation of new infection centers)
-
Spores germinate and infect an oak tree
-
Infection spreads through the vascular system of the tree and leaf symptoms develop. Discoloration develops in the vascular tissue.
-
The tree dies in 3-6 months
-
Fungal pressure pads and spore bearing mats form under the bark in red oak species.
-
Nitidulid beetles feed on the pads and emerge with spores on their bodies.
-
Nitidulids visit fresh wounds on healthy oaks and deposit spores into the vascular system.
Bottom Cycle: Underground spread by root grafts (Expansion of the infection center)
-
Fungal spores move through the vascular system through grafted root into adjacent uninfected trees.
-
Healthy trees become infected.

Oak wilt
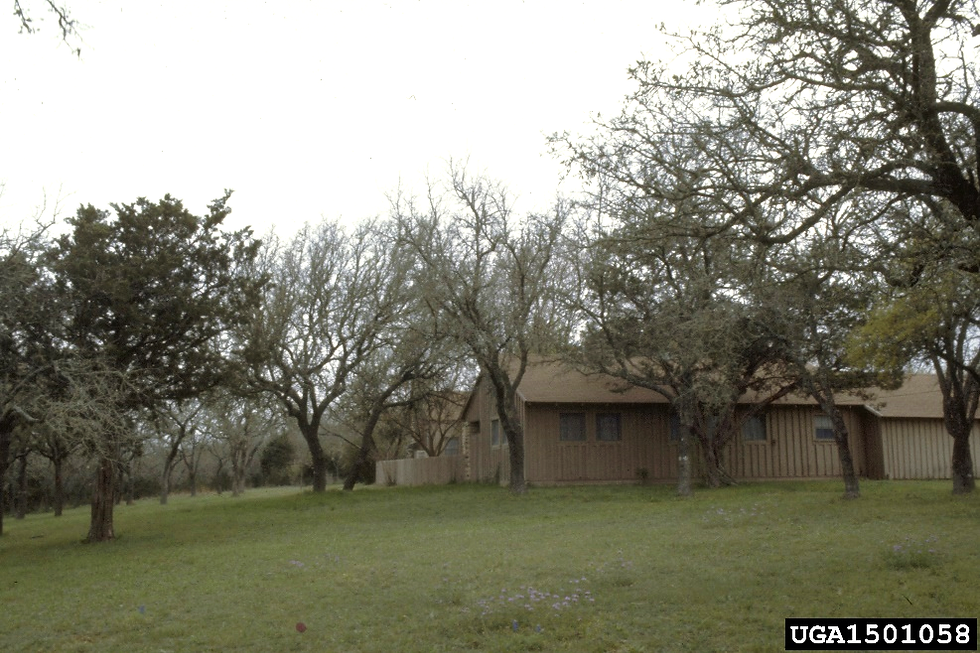
Oak Wilt
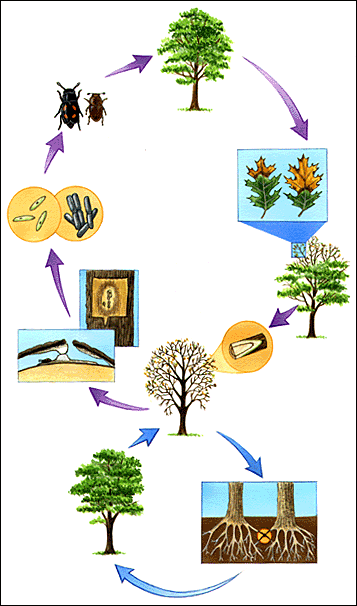
a graphic illustrating the cycle of disease

Oak wilt
Learn More
Management:
Management of oak wilt includes an integrated pest management approach by preventing new infections, diversifying the landscape, stop the spread through root connections and injection of high-value oaks with fungicides.
Root connections - To stop the spread through root connections, these connections must be severed. Install a trench at least 4 ft deep or as deep as possible to ensure all root connections are severed. Install the trench 50 to 100 feet beyond the perimeter of the infection centers and then uproot all trees within the 100-ft barrier, except those that have been treated with fungicide. The trench should be installed 50 feet away when controlling oak wilt in the mid-west and 100 feet for treatments in Texas. The trenches can be installed with a variety of equipment, excavator, rock saw, vibratory plow and bulldozer with ripper bar.
Chemical control – propiconazole is the principle fungicide for treatment against oak wilt. This chemical is injected by tree injection that involves intravascular injections with positive pressure to force the chemical through the vascular system of the tree. This injection of propiconazole into one tree does not offer protection to neighboring trees and must be applied to each high-value tree.
Pruning – limit pruning in the spring from about February to June or July depending on your area. This will prevent the nitidulid beetles from being attracted to the fresh wounds and starting a new infection in healthy trees. The least hazardous periods for pruning are during the coldest days in the winter and extended hot periods in mid to late summer. Regardless of when pruning occurs, all pruning wounds should be treated immediately with paint to prevent exposure to contaminated nitidulid beetles.
Palm Wilt
Status: Non-Native
Hosts (in California): F oxysporum f. sp. canariensis is only found on Canary Island Date Palm (Phoenix canariensis) while F. oxysporum f. sp. palmarum primarily attacks Queen Palm (Syagrus romanzoffiana) and Mexican Fan Palm (Washingtonia robusta) and, rarely, Canary Island Date Palm and the hybrid Mule Palm (known as Butia capitata in the palm trade).
Management:
Both fungi are primarily spread through pruning and other tools. Therefore, the main means of preventing spread is through disinfecting all tools used on palm trees between use. Water and soil movement can also spread the disease so minimal movement of these materials is important. There is no cure for the disease so infected palms should be entirely removed, including the root bole, and properly disposed of either by incineration or by burying in a landfill. Infected materials should not be sent to waste recycling programs since they can still spread the disease. Only palms not shown to be susceptible to the diseases should be planted back in the impacted sites.
Significance:
Palm wilt disease is a recent introduction to California. It is quite extensive in Florida and coastal Texas and has been found in Arizona.
Infected trees can live for months or even for over a year but the disease is ultimately fatal. F. oxysporum f. sp. palmarum can kill a palm within just a few months. The fungus affects the flow of water and nutrients through the main stem.
Infected trees show leaf browning and dieback beginning in the lower crown and moving up the tree until just the top spear leaves are left alive. Leaves often turn brown first on one side only with the petiole affected on only one side before the entire leaf dies. Within the trunk dark pink to orange discoloration turns brown and then black and eventually encircles the entire stem. Symptoms are often enough to identify the disease without isolation and identification of the fungi.
There is no cure for the disease and infected palms will eventually die.
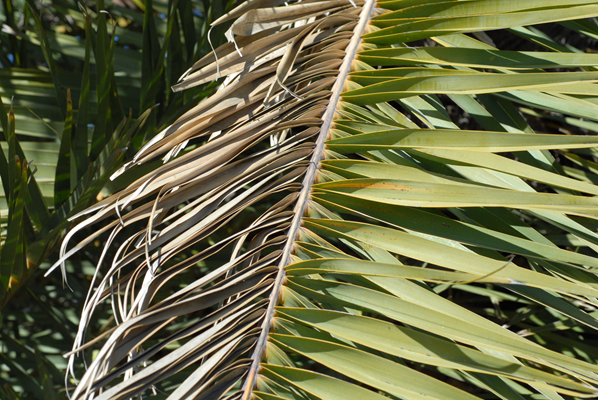
Palm frond showing wilt primarily on one side before it spreads to both sides
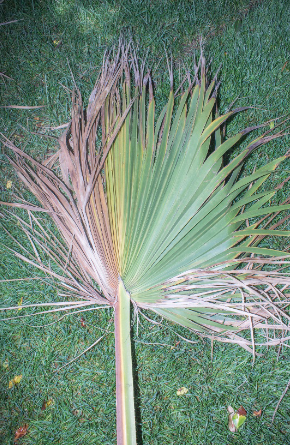
Mexican fan palm frond with one sided dieback

Palm wilt
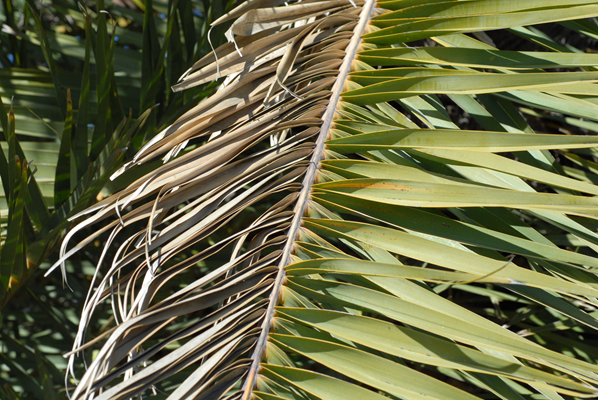
Palm frond showing wilt primarily on one side before it spreads to both sides
Learn More
Recommended Literature
Hodel, D.R. and P. Santos. 2019. Fusarium wilt of queen palm and Mexican fan palm: a devastating, new palm disease in California. PalmArbor 2:1-17.
Hodel, D.R. and P. Santos. 2020. The new fusarium wilt disease of palms in California now confirmed on Mexican fan palm. PalmArbor 14:1-23.
Phytophthora
Phytophthora lateralis
Phytophthora cinnamomi
Phytophthora cambivora
Phytophthora cactorum
Status: Both native and non-native
Location: throughout California
Hosts killed by this pathogen in California: a wide variety of trees, shrubs, and herbaceous plants
Management:
The plant disorders caused by soilborne Phytophthora species are often called “diseases of the site” because once these pathogens are established in the soil, the only way to remove them is to thoroughly fumigate the soil with chemicals that also destroy beneficial soil biota. Some success has been found with applying phosphonate fungicides as a soil drench to provide resistance to native tree and shrub species susceptible to Phytophthora cinnamomi in Australia. Since many soilborne Phytophthora species are major pests of agricultural crops, specific fungicide applications and intensive cultural practices have been developed to manage these pests in those crops. Otherwise, management of these soilborne pathogens depends largely on preventing their spread to new, uninfested areas by delineating infested areas; implementing cleanliness measures to limit the human-assisted spread of the pathogens on footwear, tools, and vehicles; and designing clean plant production systems to limit the inadvertent spread of Phytophthora species on plants grown for landscaping and ecological restoration.
The exception to the paragraph above is Phytophthora lateralis, which causes Port Orford-cedar root disease in northwestern California and southwestern Oregon. This pathogen has received intensive study leading to the development of management recommendations with a higher likelihood of success than in the case of more generalist pathogens in this genus. Depending on the management goals, geographic area of activity, and managing agency, these recommendations include implementing specific cleanliness measures; clearing Port Orford-cedar from infested sites for a period of time; removing small Port Orford-cedar regeneration from roadsides and other areas where the pathogen can easily reach the small trees to initiate new infections; and deploying known disease-resistant stock for reforestation within the natural range of Port Orford-cedar.
Highlights: Phytophthora species resemble fungi in their growth habit and production of infective spores. However, they belong a group of organisms called oomycetes, which belong in a diverse kingdom of life called Stramenopila. Other stramenopile organisms include diatoms, brown algae such as kelp, and the organisms that produce downy mildews of crops such as soybeans, hops, grapes, and squash. One way in which Phytophthora species differ from fungi is in their production of spores that can swim in water by means of flagella (all fungal spores are non-motile and get from place to place passively). Phytophthora species are commonly called “water molds” because their survival and reproduction is dependent on the presence of free water. Although many of them can produce resistant, thick-walled spores that can survive in roots or soil for long periods of time under unfavorable environmental conditions, they require at least a thin film of water to grow and reproduce. When these conditions appear—for example, during flooded conditions in the soil—Phytophthora species can produce hundreds of thousands of infective spores in a very short period of time. They are well-adapted to boom-and-bust environmental conditions.
There are around 200 known Phytophthora species, not all of which have described and named, and it is likely that many more are undiscovered. Many of these are plant pathogens, and many infect roots in roughly the same ways and produce similar symptoms. The four listed above are some of the most prominent in California.
P. lateralis was introduced from its native habitat (Taiwan) into North America in the 1920s, infecting planted Port Orford-cedars; from the point of introduction in the Pacific Northwest, the pathogen slowly spread into the native range of the tree in southwest Oregon and northwest California. By the end of the first decade of the 2000s it had become established in the southernmost and easternmost major drainages that support populations of Port Orford-cedar (southernmost: the Trinity and Mad River drainages in Humboldt County; easternmost: the upper Sacramento River drainage in Siskiyou County).
P. cinnamomi was first noted in California in the early 2000s causing dramatic mortality of a rare manzanita species, Arctostaphylos myrtifolia (Ione manzanita), in the Sierra Nevada foothills. Subsequently, it was noted in southern California on native oak species and then along the north coast associated with mortality in a diverse set of tree and shrub species including bishop pine, tanoak, madrone, bay laurel, rhododendron, chinquapin, bigleaf maple, and Douglas-fir.
P. cambivora has been associated with chinquapin mortality in roughly the same area where P. lateralis is active (northwest California/southwest Oregon). However, it has a wider host range, having also been associated with 30 other tree genera from 19 families. It has been detected throughout California and has probably been present and spreading for well over a century. P. cactorum is similar; it is very well-known as a pathogen of various agricultural crops including many fruit and nut trees. Genetic information indicates that, unlike the other Phytophthora species in this list, P. cactorum may be native to California soils.
Other soilborne Phytophthora species of particular concern in California include P. tentaculata and P. quercina. P. tentaculata has been detected on native plant species, including sticky monkeyflower (Mimulus aurantiacus), toyon (Heteromeles arbutifolia), coffeeberry (Frangula californica), and sage (Salvia spp.), that were grown and subsequently outplanted as part of native habitat restoration projects. P. quercina, which has been associated with oak decline in continental Europe, was detected in California on recently planted valley oak (Quercus lobata). The more researchers and land managers look for these soilborne pathogens in California landscapes, the more they find, making clean plant-growing practices and the development of rapid detection methods high priorities for forestry, agricultural, and restoration sectors.
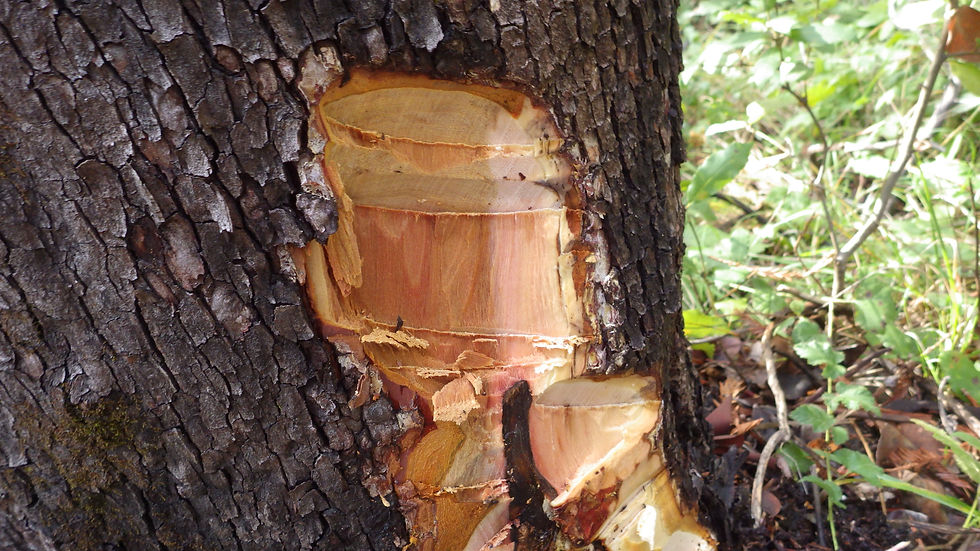
Canker (area of dead stem tissue) on a madrone tree associated with Phytophthora cinnamomi and Phytophthora cactorum at a site in Santa Cruz County. Dead tissue is darker brown and on left, while still-living tissue is lighter in color and on right
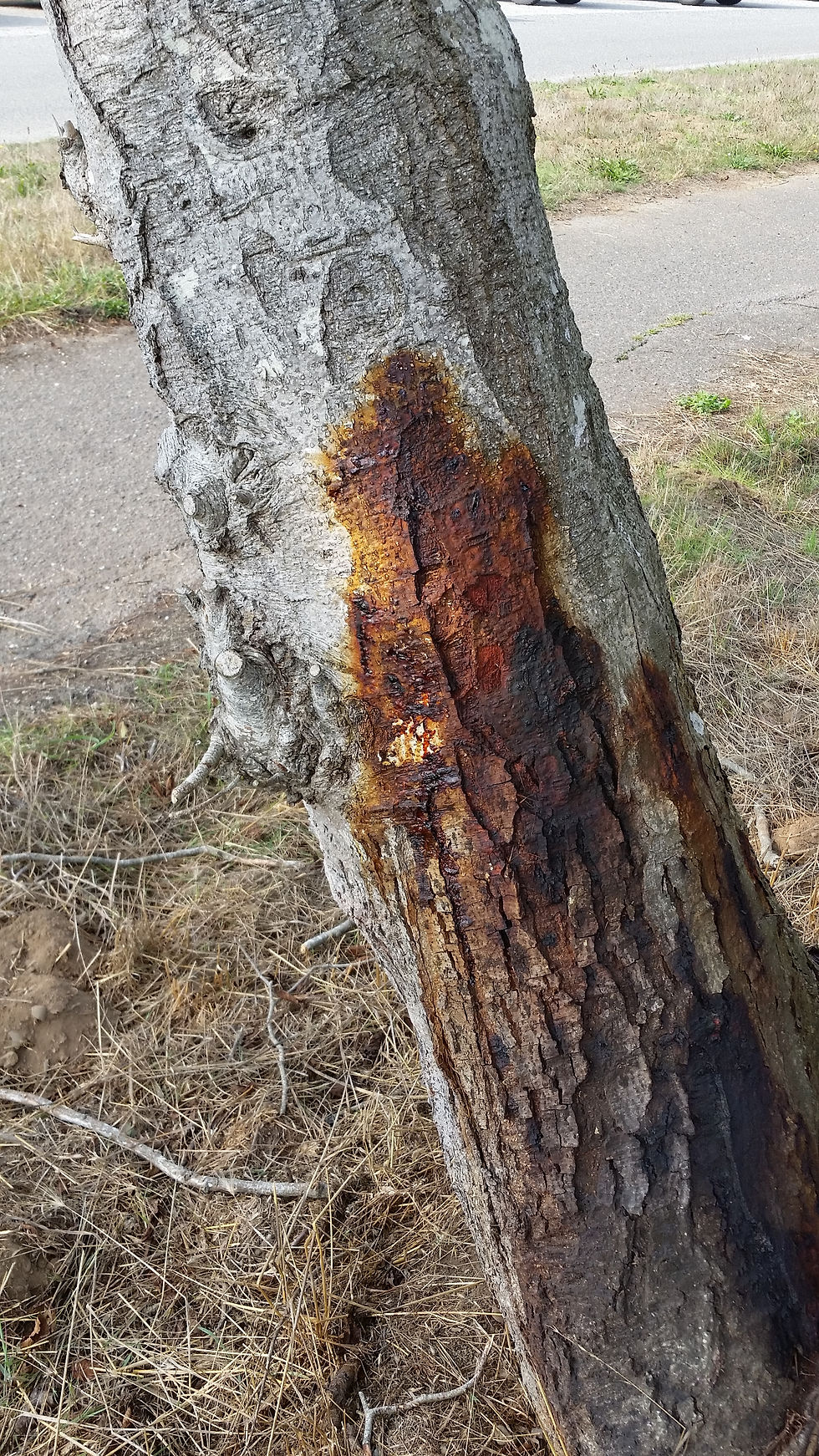
Bleeding canker of red alder caused by Phytophthora siskiyouensis in Humboldt County

Phytophthora cinnamomi
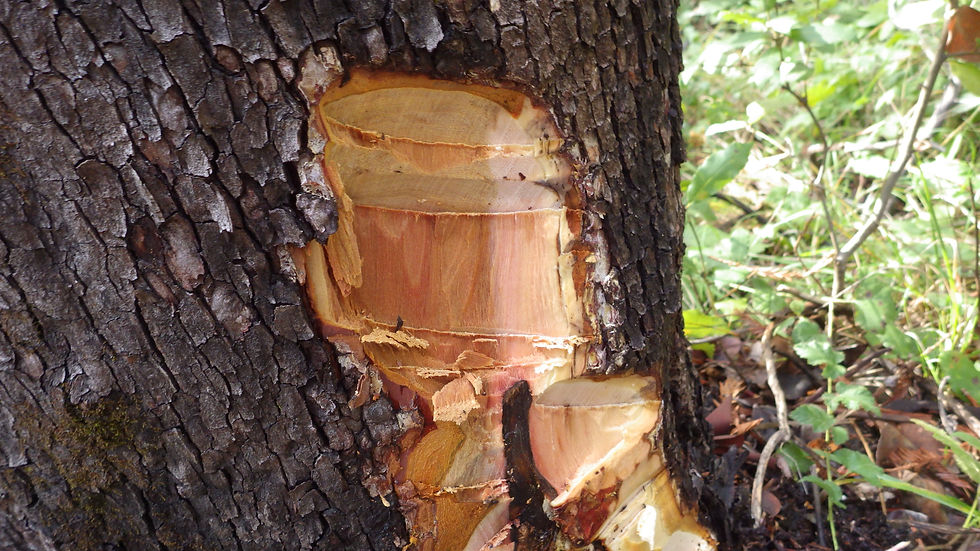
Canker (area of dead stem tissue) on a madrone tree associated with Phytophthora cinnamomi and Phytophthora cactorum at a site in Santa Cruz County. Dead tissue is darker brown and on left, while still-living tissue is lighter in color and on right
Learn More
Recommended Literature
Betlejewski, F., Casavan, K.C., Dawson, A., Goheen, D.J., Mastrofini, K., Rose, D.L., and White, D.E., eds. 2003. A Range-wide Assessment of Port-Orford-Cedar (Chamaecyparis lawsoniana) on Federal Lands. Portland, OR and Vallejo, CA: USDA Forest Service and USDI Bureau of Land Management. 182 pp. Available at https://www.fs.usda.gov/Internet/FSE_DOCUMENTS/stelprdb5316517.pdf.
Frankel, S.J., Alexander, J., Benner, D., Hillman, J., and Shor, A. 2020. Phytophthora pathogens threaten rare habitats and conservation plantings. Sibbaldia 18: 53-65. https://journals.rbge.org.uk/rbgesib/index
Frankel, S.J., Alexander, J.A., Benner, D., and Shor, A. 2018. Responding to inadvertent Phytophthora introductions in California restoration areas. California Agriculture 72(4), pp. 205 -207.
Garbelotto, M., Hüberli, D., and Shaw, D. 2007. First report on an infestation of Phytophthora cinnamomi in natural oak woodlands of California and its differential impact on two native oak species. Plant Disease 90(5), https://doi.org/10.1094/PD-90-0685C.
Goheen, D.J., Mallams, K., Betlejewski, F., and Hansen, E.M. 2012. Effectiveness of vehicle washing and roadside sanitation in decreasing spread potential of Port-Orford-cedar root disease. Western Journal of Applied Forestry 27(4), pp. 170-175.
Saavedra, A., Hansen, E.M., and Goheen, D.J. 2007.
Phytophthora cambivora in Oregon and its pathogenicity to Chrysolepis chrysophylla. Forest Pathology 37, pp. 409-419.
Sena, K., Crocker, E., Vincelli, P., and Barton, C. 2018. Phytophthora cinnamomi as a driver of forest change: Implications for conservation and management. Forest Ecology and Management 409, pp. 799-807.
Sims, L.L. and Garbelotto, M. 2021. Phytophthora species repeatedly introduced in Northern California through restoration projects can spread into adjacent sites. Biological Invasions, https://doi.org/ 10.1007/s10530-021-02496-6.
Swiecki, T.J., and Bernhardt, E. 2018. Best Management Practices for Preventing Phytophthora Introduction and Spread: Trail Work, Construction, Soil Import. Vacaville, CA: Phytosphere Research. Available online at: http://phytosphere.com/publications/Phytosphere_GGNPC_Soil_Phytophthora_BMPs_Jan2018.pdf.
Pitch Canker
Pitch canker is one of the most threatening tree diseases in the world today. Because Pinus radiata (Monterey pine or radiata pine) is the most widely planted forest tree in the world, its native range is a valuable genetic resource. These stands are also a unique and increasingly rare example of native vegetation in the central coast of California.
Location: California, Southeastern US, Mexico, Chili, Japan, South Korea, Italy, France, Spain, Portugal and South Africa.
Impact Significance: This non-native fungus affects many pine species and has spread rapidly after its introduction. This pathogen causes multiple branch infections and extensive crown dieback that may lead to tree mortality.
Hosts: Monterey pine (Pinus radiata), Knobcone pine (Pinus attenuata), Shore pine (Pinus contorta subspecies contorta), Allepo pine (Pinus halepensis), Bishop pine (Pinus muricata), Coulter pine (Pinus coulteri), Ponderosa pine (Pinus ponderosa), Gray pine (Pinus sabiniana), Torrey pine (Pinus torreyana) and Douglas-fir (Pseudotsuga menziesii).
Disease Cycle: The fungus that causes pitch canker produced spores that can be spread by air, soil, rain splash and/or transmitted by insects. In the southeastern US and most other areas, no vector relationships have been demonstrated to spread the pathogen and infection courts are caused from a variety of mechanical injuries, such as weather events causing wounds, pruning, etc. In California, however, there is evidence that insects vector the pathogen during exploratory feeding on trees, thereby created fresh wounds and inoculating the fungal spores at the same time. The insects that carry the fungus are engraver beetles (Ips species), twig beetles (Pityophthorus species), cone beetles (Conophthorus radiatae), and deathwatch beetles (Ernobius punctulatus).
Once the fungal spores are transmitted into the freshly created wounds, the fungus causes cankers that can girdle branches, exposed roots and the main stem of the pine trees. As a result of the obstructed water flow the tips of the girdled branches turn yellow then brown and the needle fascicles eventually fall off, leaving the branch ends bare. The fungus isn’t known to move systemically in the tree and therefore each canker is a separate and distinct infection. Resin accumulates on the branches or trunk at the site of the infection and the wood beneath is resin soaked and honey colored. The flow of resin from main stem or trunk infections can coat the bark up to several feet below the infection site. Flatten or slightly sunken cankers on the main stem usually appear after the tree already has multiple branch infections.
Fusarium circinatum can also cause seedling mortality. Seedling infections can result from inoculum being present on seed, in the soil or the litter layer. Emerging seedlings may die quickly and on older seedlings resin typically accumulates on the stem near the soil line with the characteristic honey-colored lesions beneath the bark.
Management: Resistance – some individual Monterey pines are resistant to the disease and these trees can be vegetatively propagated. Also some Monterey pines have systemic acquired resistance (SAR) to Fusarium circinatum, a phenomenon that elevates resistance to a pathogen after initial challenge by that pathogen. This can reduce the impact of pitch canker on that tree and maybe useful in providing resistance to other trees.
Prevent movement of pathogen - it is important to minimize the movement of this pathogen in to new uninfested locations. This can be done by cleaning and sterilizing tools and machinery before use on uninfected trees or in uninfected areas. Lysol™ or a 10% solution of bleach (1-part household bleach in 9 parts water) are effective sterilants.
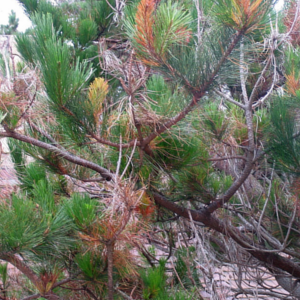
Pitch canker
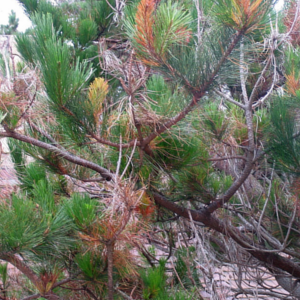
Pitch canker
Limbs and small pieces of wood from disease trees maybe chipped and mulch deposited onsite or burned. Any material that is removed should be tightly covered with a tarp during transit and taken to the nearest landfill or designated disposal facility prompt burial, chipping and composting or burning. Do not transport diseased wood out of infested counties.
Logs from diseased trees maybe used for firewood for local use, but wood should be seasoned beneath a tightly sealed clear plastic tarp to prevent the buildup of insects. Logs that are removed from the site should be taken to a designated disposal facility for prompt burial, chipping and composting or burning.
Seeds collected in pitch canker infested areas may carry the pathogen, even if they are taken from cones on apparently healthy trees. Pine seeds should not be transported out of pitch canker infested areas. To avoid such problems, do not use seed that originated within the Zone of Infestation and be sure that seedlings are disease-free.
Managing the disease in infected trees - Where trees have sustained a limited number of infections, removing symptomatic branches can effectively eliminate the disease. Of course, new infections can occur, and studies have shown that pruning doesn't slow the development of pitch canker in stands where the disease is well established. However, pruning can be used strategically to enhance the aesthetic quality of a tree and thereby delay its removal from the landscape. Limbs and small pieces of wood from disease trees maybe chipped and mulch deposited onsite or burned. Any material that is removed should be tightly covered with a tarp during transit and taken to the nearest landfill or designated disposal facility prompt burial, chipping and composting or burning. Do not transport diseased wood out of infested counties. Clean and sterilize any tools used to prune infected branches with Lysol™ or a 10% solution of bleach (1-part household bleach in 9 parts water).
Learn More
Rusts
Rusts are fungal diseases that infect many hosts, including birch, cottonwood, cypress, false cypress, fuchsia, hawthorn, juniper, pine, poplar, rhododendron, rose, and spruce.
Location: California
Impact Significance: Various
Hosts: Birch, cottonwood, cypress, false cypress, fuchsia, hawthorn, juniper, pine, poplar, rhododendron, rose, and spruce.
Biology: Fungal disease
Damage: Causes branch dieback and occasionally kills the entire plant.
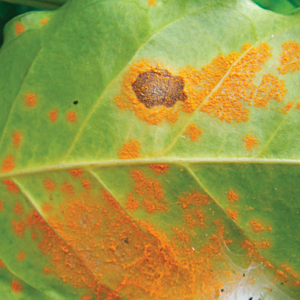
Rust
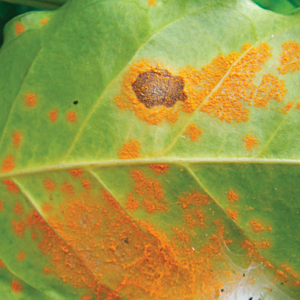
Rust
Learn More
Sudden Oak Death
Status: Non-native
Location: 16 coastal and near-coastal counties in California
Hosts killed by this pathogen in California: tanoak, coast live oak, Shreve oak, California black oak, canyon live oak, possibly several manzanita species
Hosts that carry the pathogen and/or sustain non-lethal infections on leaves or twigs: numerous native and ornamental plants in California, including herbaceous plants, woody shrubs, coniferous trees, and hardwood trees
Management:
Several attempts have been made to control Phytophthora ramorum (henceforth “P. ramorum”) in both wildland and urbanized environments. Early trials established that phosphonate fungicides are effective as a preventive treatment against infection by P. ramorum, although effectiveness may vary with tree species, tree size, and other vegetation present (e.g., proximity of target tree to infected bay laurels, density of mosses and lichens on the target tree trunk). Continued research has refined the protocols for applications of phosphonates. Additionally, judicious removal of California bay laurel, the main reservoir hosts for P. ramorum, to provide a bay laurel-free zone near susceptible oaks and tanoaks can greatly reduce the risk of infection. Both phosphonate application and bay laurel removal are most practical and cost-effective in an environment with a limited number of oak or tanoak hosts to be protected.
In wildland areas in Oregon, California, and the UK, removal of host trees with and without various additions, such as treatment of resprouting root systems with herbicide, the additions of pile burning or broadcast burning, and the removal of trees in buffer zones of varying widths around the infected trees, has been repeatedly tried. These efforts have generally succeeded in slowing the spread of the pathogen when consistently repeated year after year, but not in eradicating the pathogen. One-time treatments are generally not effective in slowing the spread, mostly because of cryptic infections in nearby, apparently asymptomatic hosts. This cryptic component highlights the necessity of continual regional- or landscape-scale monitoring efforts for successful wildland management. Monitoring is typically done by baiting local stream water for pathogen presence (earliest landscape detection); looking for tree mortality from the air; and by ground-based surveys and sample collection.
Highlights:
P. ramorum is similar to other Phytophthora species in most ways: it requires free water to complete its life cycle, it produces a number of asexual reproductive structures including sporangia, zoospores, and chlamydospores, and it reproduces sexually via stress-resistant oospores. It differs from many other Phytophthora species, however, in spreading primarily through the air column in wind-driven and splashing water, rather than through the soil. Corresponding to this means of spread, P. ramorum infects aboveground plant parts such as leaves and stems rather than roots. It also infects two primary classes of hosts. On one class it produces lethal stem infections: this class includes certain oak species and tanoaks in the U.S. and Japanese larch in the U.K. On the other class its infections cause leaf spotting, twig and shoot blight, and occasional branch cankers, but they do not generally kill the plant. Some of the latter hosts, including California bay laurel and several ornamental plants (especially in the genera Rhododendron, Camellia, Kalmia, Viburnum, and Pieris), serve as important reservoirs for the pathogen because P. ramorum readily sporulates on their foliage in great quantities. Recently, observers have noted P. ramorum causing great damage to many species of native manzanitas in California.
Sudden oak death was first noted in Marin County in the mid-1990s, and the causal pathogen had been found and named by 2000. Recent research has established that the pathogen is native to Vietnam, Japan, and probably other locations in eastern Asia. Several distinct lineages of the pathogen became established in both North American and Europe in the early 2000s and subsequently spread widely in their respective regions through hitchhiking in ornamental nursery material followed by in situ clonal reproduction and landscape-scale spread during years with heavy precipitation. During the next twenty years, detections of these separate lineages were increasingly made in close geographic proximity, suggesting the possibility in some cases that the pathogen might begin to diversify through sexual reproduction, potentially leading to novel traits or behaviors.
Learn More

The red stain of sudden oak death on the trunk of an infected oak tree

Symptoms caused by P. ramorum on California bay laurel foliage. Photo: Yana Valachovic, University of California Cooperative Extension

Sudden oak death

The red stain of sudden oak death on the trunk of an infected oak tree
Recommended Literature
Davidson, J.M., Wickland, A.C., Patterson, H.A., Falk, K.R., and Rizzo, D.M. 2005. Transmission of Phytophthora ramorum in mixed-evergreen forest in California. Phytopathology 95(5), pp. 587-596.
Davidson, J.M., Patterson, H.A., and Rizzo, D.M. 2008. Sources of inoculum for Phytophthora ramorum in a redwood forest. Phytopathology 98(8), pp. 860-866.
Hansen, E.M., Kanaskie, A., Prospero, S., McWIlliams, M., Goheen, E.M., Osterbauer, N., Reeser, P. and Sutton, W. 2008. Epidemiology of Phytophthora ramorum in Oregon tanoak forests. Canadian Journal of Forest Research 38(5), pp. 1133-1143.
He, Y., Chen, G., Cobb, R.C., Zhao, K., and Meentemeyer, R.K. 2021. Forest landscape patterns shaped by interactions between wildfire and sudden oak death disease. Forest Ecology and Management 486, 118987. https://doi.org/10.1016/j.foreco.2021.118987.
Jung, T., Scanu, B., Brasier, C.M., Webber, J., Milenković, I., Corcobado, T., Tomšovský, M., Pánek, M., Bakonyi, J., Maia, C., Bačova, A., Raco, M., Rees, H., Pérez-Sierra, A., and Jung, M.H. 2020. A survey in natural forest ecosystems of Vietnam reveals high diversity of both new and described Phytophthora taxa including P. ramorum. Forests 11, 93.
Jung, T., Jung, M.H., Webber, J.F., Kageyama, K., Hieno, A., Masuya, H., Uematsu, S., Pérez-Sierra, A., Harris, A.R., Forster, J., Rees, H., Scanu, B., Patra, S., Kudláček, T., Janoušek, J., Corcobado, T., Milenković, I., Nagy, Z., Csorba, I., Bakonyi, J., and Brasier, C.M. 2021. The destructive tree pathogen Phytophthora ramorum originates from the laurosilva forests of East Asia. Journal of Fungi 7, 226. https://doi.org/10.3390/ jof7030226.
Kasuga, T., Hayden, K.J., Eyre, C.A., Croucher, P.J.P., Schechter, S., Wright, J.W., and Garbelotto, M. 2021. Innate resistance and phosphite treatment affect both the pathogen’s and host’s transcriptomes in the tanoak-Phytophthora ramorum pathosystem. Journal of Fungi. 7(3), 198. https://doi.org/10.3390/jof7030198.
Metz, M.R., Varner, J.M., Frangioso, K.M., Meentemeyer, R.K., and Rizzo, D.M. Unexpected redwood mortality from synergies between wildfire and an emerging infectious disease. Ecology 94(10), pp. 2152-2159.
Rizzo, D.M. and Garbelotto, M. 2003. Sudden oak death: endangering California and Oregon forest ecosystems. Frontiers in Ecology and the Environment 1(4), pp. 197-204.
Rizzo, D.M., Garbelotto, M., and Hansen, E.M. 2005.
Phytophthora ramorum: integrative research and management of an emerging pathogen in California and Oregon forests. Annual Review of Phytopathology 43, pp. 309-335.
Simler, A.B., Metz, M.R., Frangioso, K.M., Meentemeyer, R.K., and Rizzo, D.M. 2018. Novel disturbance interactions between fire and an emerging disease impact survival and growth of resprouting trees. Ecology 99(10), pp. 2217-2229.
Tanoak and True Oak
Blight /Canker
Tubakia californica, Diplodia corticola,
Diaporthe nothofagi, Discula quercina
Status: Both native and non-native
Location: throughout California
Hosts damaged or killed by this pathogen in California: tanoak, golden chinquapin, coast live oak, interior live oak, California black oak, and potentially other true oaks
Management: Little is known about this disease (really a loose constellation of diseases) in California. The variety of agents found in association with symptoms would likely render one unified management prescription unrealistic. Some of the agents may intensify during drought or under other water-stress conditions, so maintaining tree vigor is likely important.
Highlights:
These maladies usually manifest as scattered individual dead leaves in oak and tanoak crowns, which may progress to substantial tree defoliation over a long period of time. In many cases they have been observed near highway corridors and bodies of water, locations that are conducive to airborne spore movement and spore persistence in the landscape.
Tubakia californica is an endophyte that causes leaf lesions and twig dieback. On California black oak and coast live oak, it causes leaf lesions that primarily follow the leaf veins, eventually enlarge to encompass larger sections of the leaf, and kill leaves and twigs. On deciduous oaks such as California black oak, infected leaves remain on the tree after turning brown in autumn. On tanoak, it causes more irregular leaf lesions but also results in widespread twig dieback. On all these species, the pathogen preferentially attacks and defoliates areas of the lower crown. Some observers have noted tree mortality after long-term infection by the pathogen, but it is not clear whether T. californica alone is responsible or whether other root, stem, or branch pathogens are acting together with it to gradually debilitate trees.
Diplodia corticola can also infect both true oaks and tanoaks. It appears capable of infecting a wider variety of woody tissues than T. californica. On mature trees, it has been isolated from twig and branch dieback as well as from bleeding cankers on boles and branches. It has also been observed causing black xylem streaking throughout the burls and root systems of killed coast live oak seedlings. It produces small, round fruiting bodies in infected, killed tissues. On tanoak, this pathogen is often associated with roughened bark, and it has occasionally been found together on the same branches as various scale insects. Whereas T. californica is suspected of being native to California, there is some evidence supporting the possibility that D. corticola is not.
Diaporthe nothofagi and Discula quercina are two other pathogens that have been isolated from tanoak twig and branch cankers in the past, but less is known about their roles. Both of these genera are known to be endophytes (microorganisms that live inside plant tissues) that can emerge in response to plant stress, especially water stress, to colonize and kill larger amounts of tissue.



Sudden oak death

Recommended Literature
Braun, U., Nakashima, C., Crous, P.W., Groenewald, J.Z., Moreno-Rico, O., Rooney-Latham, S., Blomquist, C.L., Haas, J., and Marmolejo, J. 2018. Phylogeny and taxonomy of the genus Tubakia s. lat. Fungal Systematics and Evolution 1, pp. 41-99.
Ferreira, S.L., Stauder, C.M., Martin, D.K.H., and Kasson, M.T. 2021. Morphological and phylogenetic resolution of Diplodia corticola and D. quercivora, emerging canker pathogens of oak (Quercus spp.), in the United States. Plant Disease, available at https://doi.org/10.1094/PDIS-05-20-0977-RE.
Lynch, S.C., Eskalen, A., Zambino, P., and Scott, T. 2010. First report of bot canker caused by Diplodia corticola on coast live oak, Quercus agrifolia in southern California. Plant Disease 94, p. 1510.
Lynch, S.C., Eskalen, A., Zambino, P.J., Mayorquin, J.S., and Wang, D.H. 2013. Identification and pathogenicity of Botryosphaeriaceae species associated with coast live oak (Quercus agrifolia) decline in southern California. Mycologia 105(1), pp. 125-140.
Moricca, S. and Ragazzi, A. 2008. Fungal endophytes in Mediterranean oak forests: a lesson from Discula quercina. Phytopathology 98, pp. 380-386.
Thousand Cankers
Thousand cankers disease (TCD) is a disease complex native to the western United States and primarily affects black walnut Juglans nigra. Combined activity of a fungus (Geosmithia morbida) and the walnut twig beetle (Pityophthorus juglandis).
Impact Significance:
Thousand cankers disease currently threatens millions of black walnut trees in forests and urban areas, an important species with great economic and ecological value throughout its native range.
Hosts: Black walnut, Juglans nigra
Biology: Combined activity of a fungus (Geosmithia morbida) and the walnut twig beetle (Pityophthorus juglandis).
Damage:
Fungal spores are carried and introduced into the tree by adult beetles during gallery construction. Subsequent canker development in the inner bark disrupts the flow of nutrients throughout the tree, resulting in mortality.
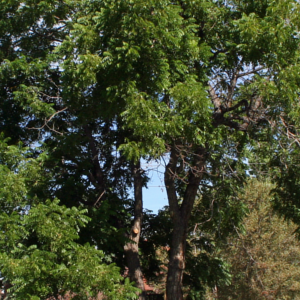
Thousand cankers oak disease
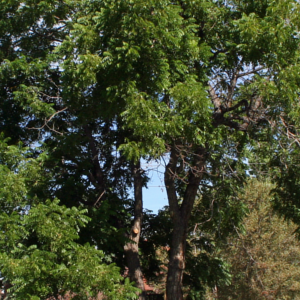
Thousand cankers oak disease
Learn More
Western Gall Rust
Status: Native
Location: Throughout California
Hosts in California: 2- and 3-needle pines including ponderosa, Jeffrey, knobcone, Coulter, gray, lodgepole (including shore), bishop, and Monterey pines
Management: Infected branches can be pruned out of trees to remove western gall rust. Because the spores of this pathogen infect the same host species from which they originate, allternate host removal is not an option as it is with some rust species. Although for management of many fungi removal of dense competing vegetation or pruning of pine stands is recommended to decrease humidity, Norlander (2008) found that such treatments were associated with the appearance of more galls, perhaps because increased airflow transported more fungal spores from tree to tree. Depending on how many trees in a stand are infected, removing these trees completely may be a viable option for some managers, as may replacing susceptible pine species with other trees that are not susceptible to this pathogen.
Highlights: This rust fungus is easily recognized by the large, spindle-shaped to spherical swellings it produces on host tree branches and stems. It can infect any size tree. Large swellings produced on main stems that cause stem deformities but do not necessarily kill the mature tree are called “hip cankers.” In some pines, especially in seedling- or sapling-size trees, the pathogen can stimulate the formation of witches’ brooms. Although may species of rust fungi produce five types of spores and must infect two different host species to complete their life cycles, western gall rust has apparently evolved beyond these complications: only three of the five spore stages are produced, and the infectious spores produced on host pine species re-infect the same pine species (in some cases, presumably, the same tree). This means that disease can build up quickly under conditions favorable to the fungus.
Western gall rust is found more often, and infestations tend to be more severe, in areas where these favorable conditions prevail. This includes humid areas near the coast, near waterbodies, in depressions on the landscape, and in densely crowded forest stands. This pathogen appears to be a “cumulative” damage factor in that over many years it gradually spreads from one part of the tree crown to another, slowly killing branches and branch tips, providing entry courts for decay fungi and bacteria, and working together with other stress-causing disease agents such as dwarf mistletoe to wear down the physiology of the aging tree. Occasionally “wave years” occur in which weather conditions are conducive to explosive pathogen reproduction and spread. As with many other pathogenic fungi, these conditions probably take place in times of repeated heavy rainfall and relatively warm temperatures (e.g., rainy springs or winters with many warm storms). Additionally, in some locations humidity may be sufficient for longer sporulation periods, leading to intensified pathogen impacts. This seems to be the case, for example, along many sections of the California coastline, where the pathogen has been observed sporulating throughout most of the summer and where it causes heavy infestations in Monterey and bishop pines.
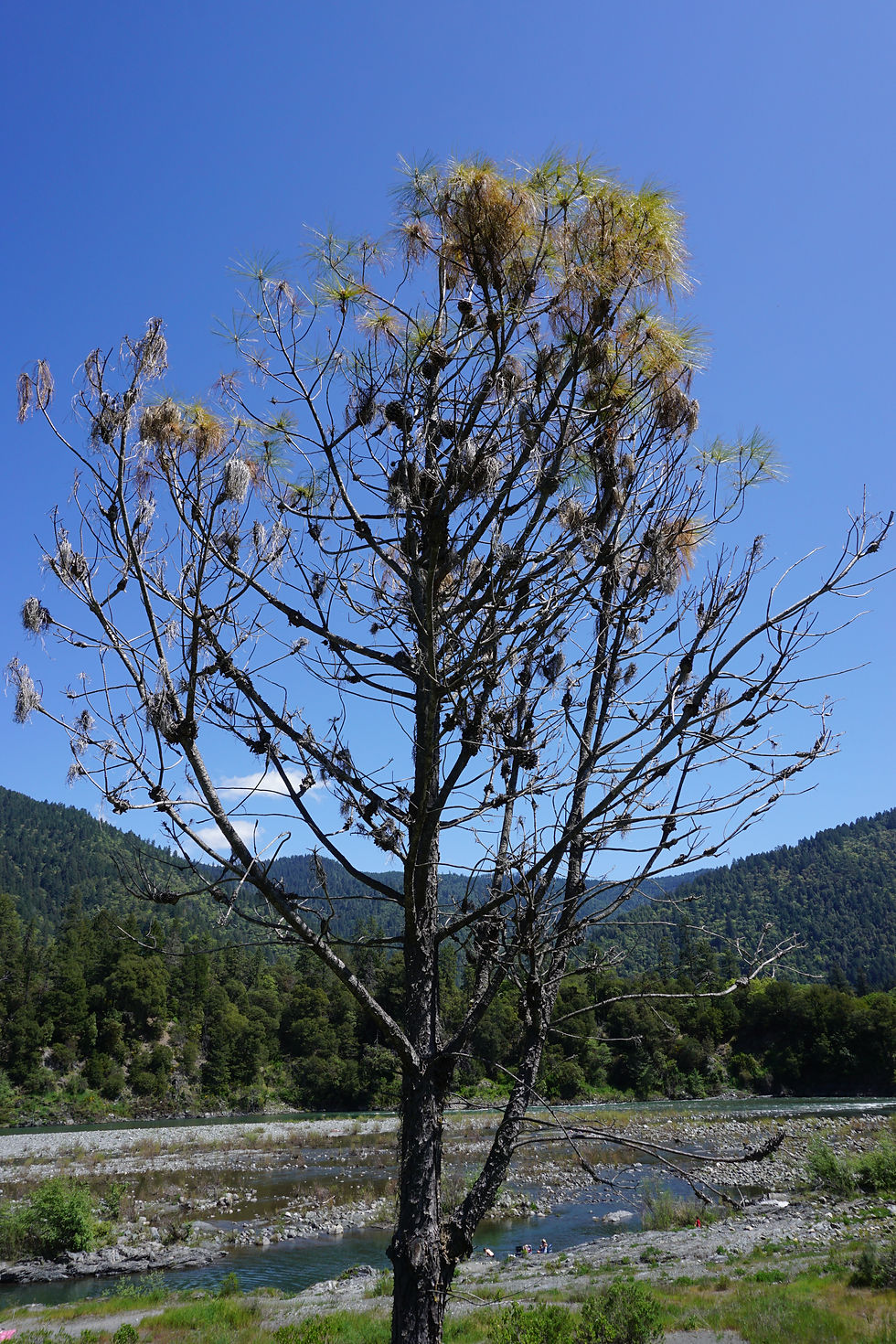
Western gall rust on ponderosa pine. Most swellings on the branches of this tree are galls, not cones
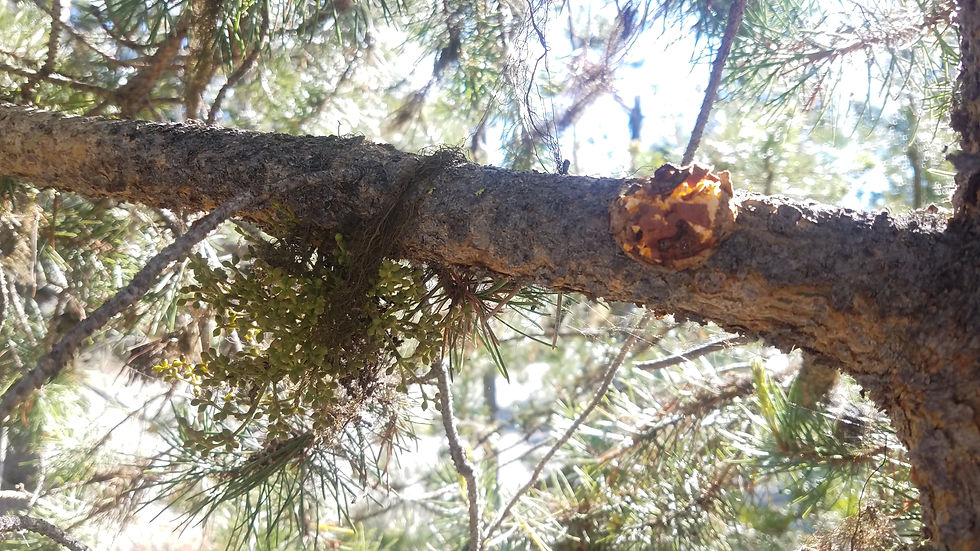
Western gall rust and dwarf mistletoe infecting a lodgepole pine branch

White pine blister rust
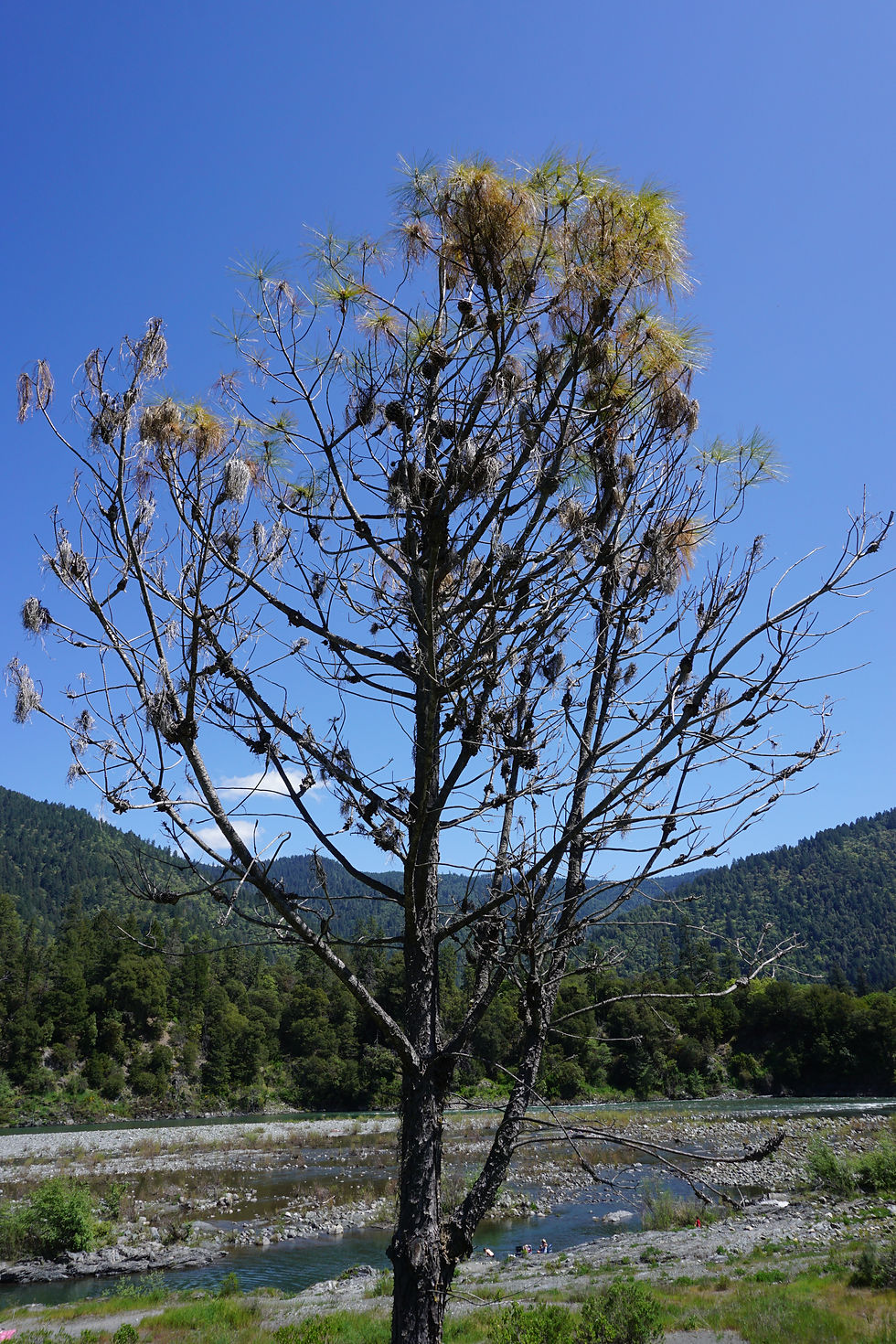
Western gall rust on ponderosa pine. Most swellings on the branches of this tree are galls, not cones
Recommended Literature
Blenis, P.V. and Duncan, I. 1987. Management implications of western gall rust in precommercially thinned lodgepole pine stands. Canadian Journal of Forest Research 27, pp. 603-608.
Byler, J.W., Cobb, F.W. Jr., and Parmeter, J.R. Jr. 1972. Effects of secondary fungi on the epidemiology of western gall rust. Canadian Journal of Botany 50(5), pp. 1061-1066.
Chang, K.-F., and Blenis, P.V. 1989. Survival of Endocronartium harknessii teliospores in a simulated airborne state. Canadian Journal of Botany 67(3), pp. 928-932.
Hopkin, A.A., Reid, J., Hiratsuka, Y., and Allen, E. 1998. Initial infection and early colonization of Pinus contorta by Endocronartium harknessii (western gall rust). Canadian Journal of Plant Pathology 10(3), pp. 221-227.
Norlander,D.W.D. 2008. Effect of Site and Silvicultural Treatment on Insect Pests and Diseases of Young Ponderosa Pine. M.S. Thesis, Oregon State University. 72 pp. available at: https://ir.library.oregonstate.edu/dspace/bitstream/1957/9139/1/Daniel%20Norlander%20Thesis.pdf.
Old, K.M. 1981. Western gall rust, a serious disease of Pinus radiata in California. Australian Forestry 44(3), pp. 178-184.
Old, K.M., Libby, W.J., Russell, J.H., and Eldridge, K.G. 1986. Genetic variability in susceptibility of Pinus radiata to western gall rust. Silvae Genetica 35(4), pp. 145-149.
van der Kamp, B.J. 1988. Temporal and spatial variation in infection of lodgepole pine by western gall rust. Plant Disease 72, pp. 787-790.
Learn More
White Pine Blister Rust
A major threat to high elevation white pines and their ecosystems is from the non-native fungus that causes the disease white pine blister rust. All North American white pines are susceptible to the rust and show low levels of resistance and high mortality rates.
Location: California, US, Canada, Asia, Europe
Impact Significance: White pine blister rust overall effect has been reducing the diversity of tree species present in many native stands and threatens the conservation and health of native ecosystems with a high component of susceptible five-needle pines.
Hosts: Western white pine (Pinus monticola), whitebark pine (Pinus albicaulis), foxtail pine (Pinus balfouriana), limber pine (Pinus flexilis ) and bristlecone pine (Pinus longaeva).
Disease Cycle: White pine blister rust has a complex life cycle that requires two hosts, a white pine and current or gooseberry plant (Ribes spp.) to complete the life cycle. Recently however, Indian paint brush (Castillija spp.) and snapdragon (Pedicularis spp.) have been discovered to be alternate hosts as well.
The rust pathogen produces 5 distinct spore stages (diagram below) and the blister rust life cycle starts in the fall when pine needles are infected with basidiospores from the alternate host (A). The basidiospore germinate, and the fungus mycelium enters the needles through stomata and grows down the needle into the branch. The fungus continues to grow and develop lengthwise and laterally in a spindle shaped pattern to produce a swollen canker (B). in the spring of the second and third year, pycnia and aecia are produced (B,C) and erupt through the bark. This causes the bark to dry and death of the inner bark occurs and this dead area enlarges over several years to become a canker. The aeciospores can survive for several months and are wind-borne for long distances (up to 500 km in the jet stream) where they infect the alternate hosts.
After a few weeks, the Ribes or other alternative hosts are infected and develop yellow spots on the upper leaf surface (E) and urediniospores are produced on the underside of the leaves (G). These spores build up and keep re-infecting the alternate host. Basidiospores are finally produced from teliospores on the underside of the alternate hosts(H) and spread to infect the pine hosts (A) to complete the disease cycle.
Blister rust is favored by cool, moist conditions, and the disease tends to be more prevalent in low-lying areas like creek bottoms, swampy areas and openings in timber stands.
All species of white pine are susceptible at all ages; however, seedlings, sapling and pole sized trees are more severely infected and die because of infection than older trees. This is mainly because young trees are closer to the ground where conditions are more favorable for infection, needles are closer to the main trunk so less time is needed for infections to move to get into the main stem and kill trees.
Management:
Quarantines and host removal – attempts were made to control blister rust by destroying all infected white pine as they were found, but the long latent period of infection deemed this method not effective. There was also the well-known program for the eradication of Ribes which received support from the federal government during the great depression. This reduced the amount of Ribes but was unsuccessful at preventing the disease from occurring.
Pruning – removing lower branches on young trees and removal of diseased branches is recommended in some areas. Pruning should occur over several years and no more than 1/3 of the branches should be removed at any one time. When cankers are closer than 4-6 inches to the trunk removal of that canker is not possible because it is probably already infected the main stem.
Rust hazard zones – these are high hazard zones that occur in cool, moist environments where the moist air settles into depressions where air drainage is poor. These areas consist of valleys, stream bottoms and opening in the forests. Generally, upper slopes and ridges are less conducive to blister rust infections than benches and valleys.
Genetic resistance - The occurrence of apparently resistant trees in areas where the rust was severe was recognized in eastern white pine as early as 1937. Surveys of heavily rusted sugar pine and western white pine stands led to selection of large numbers of apparently rust-free, and presumably resistant, trees as parent trees for disease resistance breeding programs. The programs for the western pines started about 1950. By crossing and backcrossing these parent trees and the subsequent progeny, several rust-resistant lines have been developed. These resistant trees are used to establish seed orchards and some are now producing seed for general distribution. The Region 5 Genetic Resources Program is a facility in California that screens sugar and western white pines for genetic resistance to white pine blister rust. Screening for major gene resistance (MGR) and slow rusting resistance (SRR) is occurring and reforestation efforts have taken place with rust resistant sugar pines.

White pine blister rust

White pine blister rust